The Functions, Disease-Related Dysfunctions, and Therapeutic Targeting of Neuronal Mitochondria Wiley Series on Neuropharmacology Series
Auteur : Hardwick J. Marie
Coordonnateurs : Gribkoff Valentin K., Jonas Elizabeth A.
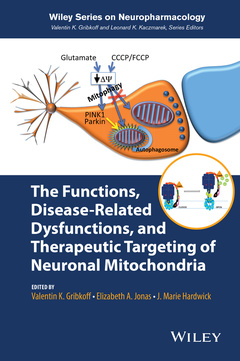
? Helps readers understand the regulation of mitochondrial cellular processes, such as substrate metabolism, energy production, and programmed versus sporadic cell death
? Offers insights on the development of strategies for targeted therapeutic approaches and potential personalized treatments
? Includes examples of mitochondrial drugs, development, and mitochondria-targeted approaches for more efficient treatment methods and further developments in the field
? Covers the model systems and approaches needed for the development of new drugs for the central nervous system to provide potential modern therapeutics for neurodegenerative disorders
Contributors xiv
Preface xviii
Section I Mitochondrial Structure and Ion Channels 1
1 Mitochondrial Permeability Transition: A Look From a Different Angle 3
Nickolay Brustovetsky
1.1 Regulation of Intracellular Calcium in Neurons 3
1.2 Calcium Overload and Mitochondrial Permeability Transition 4
1.3 The Mitochondrial Transition Pore 8
1.3.1 Evidence for ANT and VDAC as Components of the PTP 8
1.3.2 Alternative Hypotheses of mPTP Composition 17
Acknowledgments 22
References 22
2 The Mitochondrial Permeability Transition Pore, the c]Subunit of the F1FO AT P Synthase, Cellular Development, and Synaptic Efficiency 31
Elizabeth A. Jonas, George A. Porter, Jr., Gisela Beutner, Nelli Mnatsakanyan and Kambiz N. Alavian
2.1 Introduction 32
2.2 Mitochondria at the Center of Cell Metabolism and Cell Death 32
2.3 Mitochondrial Inner Membrane Leak: Regulator of Metabolic Rate and Uncoupling 32
2.4 Mitochondrial Inner Membrane Channels and Exchangers are Necessary for Ca2+ Cycling and Cellular Ca2+ Dynamics 33
2.5 Mitochondrial Inner and Outer Membrane Channel Activity Regulates Ca2+ Re]Release from Mitochondria after Buffering 34
2.6 Bcl]2 Family Proteins Regulate Pathological Outer Mitochondrial Membrane Permeabilization (MOMP) 35
2.7 Pathological Inner Membrane Depolarization: Mitochondrial Permeability Transition 36
2.8 The Quest for an Inner Membrane Ca2+]Sensitive Uncoupling Channel: The PT Pore 37
2.8.1 Electrophysiologic Properties of the mPTP 37
2.8.2 Characterization of a Molecular Complex Regulating the Pore 39
2.8.3 Bcl]xL Regulates Metabolic Efficiency by Binding to the β]Subunit of the ATP Synthase 39
2.8.4 CypD Binds to ATP Synthase and Regulates Permeability Transition 40
2.8.5 PT Activity Regulates Cardiac Development 41
2.8.6 Regulatory Molecules Do Not Form the Pore of mPTP 42
2.9 The mPTP: A Molecular Definition 43
2.9.1 The c]Subunit of F1FO ATP Synthase Comprises the PT Pore 43
2.9.2 The c]Subunit of ATP Synthase Creates the High Conductance mPTP Pore 45
2.9.3 F1 Regulates Biophysical Characteristics of the Purified c]Subunit 45
2.9.4 Structural Location of the Pore within the c]Subunit Ring 48
2.10 Closing of the mPTP May Enhance Mitochondrial Metabolic Plasticity and Regulate Synaptic Properties in
Hippocampal Neurons 49
2.11 mPTP Opening Correlates with Cell Death in Acute Ischemia, ROS Damage, or Glutamate Excitotoxicity 49
2.12 Pro]Apoptotic Proteolytic Cleavage Fragment of Bcl]xL Causes Large Conductance Mitochondrial Ion Channel Activity Correlated with Hypoxic Synaptic Failure: Outer Mitochondrial Channel Membrane Activity Alone or mPTP? 51
2.13 S ynaptic Responses Decline during Long]Term Depression in Association with Bcl]2 Family]Regulated Mitochondrial Channel Activity 52
2.14 S ynapse Loss During Neurodegenerative Disease May Require Mitochondrial Channel Activity 53
2.15 Conclusions 54
Acknowledgments 55
References 55
3 Mitochondrial Channels in Neurodegeneration 65
Pablo M. Peixoto, Kathleen W. Kinnally and Evgeny Pavlov
3.1 Introduction 65
3.2 Mitochondrial Channels in the Healthy Neuron 66
3.2.1 Voltage Dependent Anion]Selective Channel is the Food Channel 66
3.2.2 Protein Import Channels 67
3.2.3 Mitochondrial Ca2+ Channels 74
3.2.4 Mrs2 – Mg2+ Channel 75
3.2.5 Mitochondrial K+ Channels 76
3.2.6 Mitochondrial Centum Pico]Siemens 76
3.2.7 Alkaline]Induced Anion]Selective Activity and Alkaline]Induced Anion]Selective Activity 77
3.2.8 Chloride Intracellular Channels 78
3.2.9 Alternative Ion Transport Pathways 78
3.3 Mitochondrial Channels in the Dying Cell 79
3.3.1 Apoptosis 79
3.3.2 Necrosis 80
3.4 Mitochondrial Channels in Neurodegenerative Diseases 83
3.5 Conclusions 87
References 87
Section II Control of Mitochondrial Signaling Networks 101
4 Mitochondrial Ca2+ Transport in the Control of Neuronal Functions: Molecular and Cellular Mechanisms 103
Yuriy M. Usachev
4.1 Introduction 103
4.2 Physiological and Pharmacological Characteristics of Mitochondrial Ca2+ Transport in Neurons 106
4.3 Molecular Components of Mitochondrial Ca2+ Transport in Neurons 110
4.4 Mitochondrial Ca2+ Signaling and Neuronal Excitability 114
4.5 Mitochondrial Ca2+ Cycling in the Regulation of Synaptic Transmission 115
4.6 Mitochondrial Ca2+ Transport and the Regulation of Gene Expression in Neurons 118
4.7 Future Directions 119
Acknowledgments 120
References 120
5 A MP]Activated Protein Kinase (AMPK) as a Cellular Energy Sensor and Therapeutic Target for Neuroprotection 130
Petronela Weisová, Shona Pfeiffer and Jochen H. M. Prehn
5.1 Introduction 130
5.1.1 AMPK Expression, Structure, and Activity Regulation in Brain 131
5.1.2 Other Roles for AMPK 135
5.1.3 AMPK in Neurological Diseases and Neurodegeneration 137
5.2 Conclusion and Future Perspectives 139
References 139
6 HDA C6: A Molecule with Multiple Functions in Neurodegenerative Diseases 146
Yan Yan and Renjie Jiao
6.1 Introduction 146
6.2 Molecular Properties of HDAC6 147
6.2.1 Classes of the HDAC Family 147
6.2.2 HDAC6 149
6.3 HDAC6 and Neurodegenerative Diseases 151
6.3.1 HDAC6 and Elimination of Proteotoxicity in Neurodegenerative Diseases 152
6.3.2 HDAC6 and Autophagic Clearance of Dysfunctional Mitochondria 156
6.4 Perspectives 158
References 159
7 Neuronal Mitochondrial Transport 166
Adam L. Knight, Yanmin Chen, Tao Sun and Zu]Hang Sheng
7.1 Introduction 166
7.2 Complex Motility Patterns of Axonal Mitochondria 168
7.3 Mechanisms of Mitochondrial Transport 169
7.3.1 Kinesin Motors and Anterograde Transport 169
7.3.2 Dynein Motors and Retrograde Transport 171
7.3.3 Interplay of Opposing Motor Proteins 172
7.4 Mechanisms of Axonal Mitochondrial Anchoring 172
7.5 Regulation of Mitochondrial Transport by Synaptic Activity 173
7.6 Mitochondrial Transport and Synaptic Transmission 174
7.7 Mitochondrial Transport and Presynaptic Variability 175
7.8 Mitochondrial Transport and Axonal Branching 176
7.9 Mitochondrial Transport and Mitophagy 178
7.10 Conclusions and New Challenges 180
Acknowledgments 180
References 181
8 Mitochondria in Control of Hypothalamic Metabolic Circuits 186
Carole M. Nasrallah and Tamas L. Horvath
8.1 Introduction 186
8.2 Yin]Yang Relationship between Components of Hypothalamic Feeding and Satiety Circuits 187
8.3 Mitochondria and Their Dynamics 189
8.4 Metabolic Principles of Hunger and Satiety Promotion: Mitochondria in Support of Fat Versus Glucose Utilization 191
8.5 Mitochondria Dynamics and Cellular Energetics 193
8.5.1 Fission and Fusion of Mitochondria in Hypothalamic Feeding Circuits 194
8.6 Mitochondrial Dysfunction and Metabolic Disorders 196
8.7 Conclusions 197
References 197
9 Mitochondria Anchored at the Synapse 203
George A. Spirou, Dakota Jackson and Guy A. Perkins
9.1 Introduction 203
9.2 Calibrated Positioning of Mitochondria 204
9.3 Mitochondria and Crista Structure 206
9.4 Adhering Junctions and Linkages to the Cytoskeleton 208
9.5 Linkages of the OMM to the Mitochondrial Plaque and Reticulated Membrane 210
9.6 Functions of the Organelle Complex 211
9.7 MACs and Filamentous Contacts: A Continuum of Structure? 213
Acknowledgments 214
References 214
Section III Defective Mitochondrial Dynamics and Mitophagy 219
10 Neuronal Mitochondria are Different: Relevance to Neurodegenerative Disease 221
Sarah B. Berman and J. Marie Hardwick
10.1 Introduction 221
10.2 Mitochondrial Dynamics in Neurons and Neurodegenerative Disease 222
10.2.1 Quantifying Mitochondrial Dynamics 222
10.2.2 Mutations and Toxins Alter Mitochondrial Dynamics in Neurological Disease 223
10.3 Triggering Mitophagy in Neurons versus Other Cell Types 226
10.3.1 Parkin Mitophagy Pathway Disease Genes 226
10.3.2 Metabolic States of Neurons Modulate Mitophagy Induction 227
10.3.3 Neurons Distinguish between Different Types of Mitochondrial Damage 228
10.4 BCL]xL: The Guardian of Mitochondria 231
10.4.1 BCL]xL Regulates Mitochondrial Dynamics and Neuronal Activity 231
10.4.2 BCL]xL Regulates Mitochondrial Energetics 232
Acknowledgments 233
References 233
11 PINK1 as a Sensor for Mitochondrial Function: Dual Roles 240
Erin Steer, Michelle Dail and Charleen T. Chu
11.1 Introduction 240
11.2 PINK1 Promotes Mitochondrial Function 241
11.3 Healthy Mitochondria Import and Process PINK1 244
11.3.1 Localization and Processing of PINK1 Depends on an Intact ΔΨm 244
11.4 Accumulation of Full Length]PINK1 as a Sensor of Mitochondrial Dysfunction 245
11.5 Cytosolic PINK1 as a Sensor for Mitochondrial Function 247
11.5.1 Cytosolic PINK1 Suppresses Cell Death and Autophagy/Mitophagy 247
11.5.2 Cytosolic PINK1 Promotes Neurite Extension and Cell Survival 248
11.6 PINK1 and Mitochondrial Dynamics 248
11.7 Dual Roles for PINK1 as a Sensor of Mitochondrial Function and Dysfunction 249
References 249
12 A Get]Together to Tear It Apart: The Mitochondrion Meets the Cellular Turnover Machinery 254
Gian]Luca McLelland and Edward A. Fon
12.1 Mitochondrial Quality Control in Neurodegeneration 254
12.2 An Overview of the Ubiquitin]Proteasome System 255
12.3 Activities of the Cytosolic Proteasome at the Outer Mitochondrial Membrane 256
12.4 The Turnover of Whole Mitochondria by Mitophagy 260
12.5 Proteasomes and Phagophores Converge in the PINK1/parkin Pathway 261
12.6 Implications of PINK1]/Parkin]Dependent Mitophagy in the Brain and in PD 265
12.7 Emerging Mitochondrial Quality Control Mechanisms 267
References 268
13 Mitochondrial Involvement in Neurodegenerative Dementia 280
Laura Bonanni, Valerio Frazzini, Astrid Thomas and Marco Onofrj
13.1 Introduction 280
13.2 Mitochondrial Dysfunction in Alzheimer Disease 281
13.3 Mitochondrial Dysfunction, Bioenergetic Deficits, and Oxidative Stress in AD 282
13.4 Mitochondrial Fragmentation in AD 283
13.5 S ynaptic Mitochondria in AD 283
13.6 Mitochondrial Dysfunction and Cationic Dyshomeostasis in AD 284
13.7 Mitochondrial Dysfunction in DLB 286
13.8 LRRK2 Mutations, Mitochondria and DLB 287
13.9 Akinetic Crisis in Synucleinopathies is Linked to Genetic Mutations Involving Mitochondrial Proteins 287
13.10 Conclusions 289
References 289
Section IV Mitochondria-Targeted Therapeutics and Model Systems 295
14 Neuronal Mitochondria as a Target for the Discovery and Development of New Therapeutics 297
Valentin K. Gribkoff
14.1 Neurodegenerative Disorders and the Status of Drug Discovery 297
14.2 Mitochondria as Targets for the Development of New NDD Therapies 300
14.3 The Effects of Dexpramipexole on Mitochondrial Conductances: An Example of an Approach for ALS and Other NDDs 301
14.3.1 ALS as a Therapeutic Target 301
14.3.2 Mitochondrial Dysfunction in ALS 303
14.3.3 Dexpramipexole and Bioenergetic Efficiency: Preclinical Studies 303
14.3.4 Dexpramipexole in the Clinic 309
14.4 What is the Future of a Mitochondrial Approach for NDD Therapy? 313
Acknowledgments 314
References 315
15 Mitochondria as a Therapeutic Target for Alzheimer’s Disease 322
Clara Hiu]Ling Hung, Sally Shuk]Yee Cheng, Simon Ming]Yuen Lee and Raymond Chuen]Chung Chang
15.1 Introduction 322
15.2 Mitochondrial Abnormalities and Dysfunction in Alzheimer’s Disease 323
15.2.1 Mitochondrial Morphology and Ultrastructure 323
15.2.2 Beta Amyloid, Tau, and Mitochondria 323
15.2.3 Defective Mitochondria at Synapses 325
15.2.4 Impaired Mitochondrial Dynamics 325
15.2.5 Oxidative Stress 326
15.2.6 Ca2+ Dysregulation in Mitochondria 326
15.2.7 Mitochondrial Permeability Transition Pore 327
15.3 Mitochondria as a Drug Target 327
15.3.1 Targeting Drugs to Mitochondria 327
15.3.2 Mitochondria]Targeted Antioxidants 329
15.3.3 Mitochondrial Ca2+ Pathways 330
15.3.4 Mitochondrial Permeability Transition Pore 331
15.3.5 Mitochondrial Dynamics 331
15.3.6 Mitochondrial Metabolism 332
15.3.7 Mitochondrial Biogenesis 332
15.3.8 Limitations of Mitochondrial]Targeted Drugs 333
15.4 Conclusions 333
Acknowledgments 333
References 334
16 Mitochondria in Parkinson’s Disease 339
Giuseppe Arena and Enza Maria Valente
16.1 Introduction 339
16.2 Role of Mitochondria in Sporadic PD 340
16.2.1 Complex I Deficiency and mtDNA Defects 340
16.2.2 Oxidative Stress and ROS Production 341
16.3 Mitochondrial Dysfunction in Monogenic PD 342
16.3.1 Autosomal Dominant PD 343
16.3.2 Autosomal Recessive PD 346
16.4 Conclusions 350
References 351
17 Therapeutic Targeting of Neuronal Mitochondria in Brain Injury 359
Heather M. Yonutas, Edward D. Hall and Patrick G. Sullivan
17.1 Introduction 359
17.2 Mitochondria Bioenergetics 360
17.3 Traumatic Brain Injury 363
17.3.1 Models of TBI 364
17.3.2 Secondary Injury Cascade of TBI 366
17.4 Pharmaceutical Interventions 370
17.4.1 Targeting Mitochondrial Dysfunction 370
17.4.2 Targeting Oxidative Stress 371
17.4.3 Interventions with Multiple Targets 372
17.5 Conclusion 372
References 373
18 The Use of Fibroblasts from Patients with Inherited Mitochondrial Disorders for Pathomechanistic Studies and Evaluation of Therapies 378
Devorah Soiferman and Ann Saada
18.1 Introduction 378
18.1.1 Identification of Mitochondrial Disorders 380
18.1.2 Pathomechanism of Mitochondrial Disorders 381
18.1.3 Treatment of Mitochondrial Disorders 382
18.1.4 Models of Mitochondrial Disorders 383
18.2 Pathomechanistic Studies of Mitochondrial Disorders in Patients’ Fibroblasts 385
18.2.1 Reduced Cellular ATP 385
18.2.2 Increased Oxidative Stress 386
18.2.3 Reduction of Mitochondrial Membrane Potential 386
18.2.4 Disruption of Calcium Homeostasis 386
18.2.5 Coenzyme Q10 Deficiency 387
18.2.6 Mitochondrial Dynamics and Mitophagy 387
18.3 Evaluation of Therapeutic Options Using Patient Derived Fibroblasts 388
18.3.1 Pharmacological Approaches 388
18.3.2 Genetic Manipulation 391
18.4 Conclusion 392
Acknowledgments 393
References 393
Index 399
Valentin Gribkoff is an Associate Professor Adjunct in the Department of Internal Medicine at Yale University School of Medicine and is a founding member of The Northwoods Group, a biotech consulting and development consortium. He previously co-edited Structure, Function and Modulation of Neuronal Voltage-Gated Ion Channels (Wiley, 2009) and is a co-editor of the Wiley Series on Neuropharmacology.
Elizabeth Jonas is an Associate Professor at the Departments of Internal Medicine, Section of Endocrinology, and Neurobiology at Yale University School of Medicine.
J. Marie Hardwick is the David Bodian Professor of Molecular Microbiology and Immunology at The Johns Hopkins Bloomberg School of Public Health.
Date de parution : 10-2015
Ouvrage de 448 p.
16x23.6 cm