Brain Slices, Softcover reprint of the original 1st ed. 1984
Langue : Anglais
Auteur : Dingledine Raymond
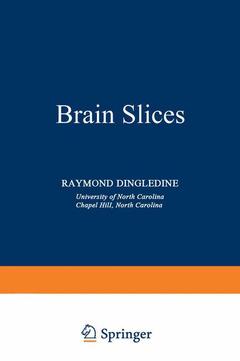
In little less than a decade brain slices have gained prominence among neurobiologists as appropriate tools to study cellular electrophysiolog ical aspects of mammalian brain function. The purpose of this volume is to present in some detail several inquiries in the brain sciences that have benefited greatly by the use of brain slices. The book is directed primarily toward advanced students and researchers wishing to evaluate the impact these in vitro preparations of the mammalian brain are having on neurobiology. The term brain slice has come to refer to thin (100-700 j. Lm) sections of a brain region prepared from adult mammals and maintained for many hours in vitro, for either electrophysiological or biochemical stud ies. In addition to good accessibility, slices feature relatively intact syn aptic connections that allow a variety of experiments not feasible with standard in vivo or tissue culture preparations. Certain electrophysiol ogical studies once practical only with invertebrate models are becoming routine with mammalian brain slices. The ability to perform both bio chemical and electro physiological experiments on the same piece of CNS tissue provides additional bright prospects for future research. Although most of the electrophysiological studies have dealt with hippocampal slices, it should be evident from this book that slice methodology is not limited to the hippocampus. The Appendix, "Brain Slice Methods," is a multiauthored treatment of the technical aspects of brain slice work, collected into one document.
Introduction: Cerebral Subsystems as Biological Entities.- 1. Comparative Electrobiology of Mammalian Central Neurons.- 1. Introduction.- 2. The Generalized Neuron.- 2.1. The Axon.- 3. Electrophysiology of the Neuronal Somata.- 3.1. The Na Conductances.- 3.2. Ca Conductances.- 3.3. The K Conductances.- 4. Dendritic Electrophysiology.- 5. Discussion.- 6. References.- 2. Passive Electrotonic Structure and Dendritic Properties of Hippocampal Neurons.- 1. Introduction.- 1.1. Why Develop Models of Neurons?.- 1.2. Hippocampal Slices as a Substrate for Theoretical Models.- 2. Electrotonic and Circuitry Models—An Overview of Methods.- 2.1. Network Model.- 2.2. Continuous Cable Models.- 2.3. Compartmental Models.- 2.4. Neuronal Circuit Models.- 3. Models Constructed with Data from Work on Hippocampal Slices.- 3.1. Continuous Cable Models.- 3.2. The Compartmental Model.- 3.3. Neuronal Circuitry Model.- 3.4. Overview of Hippocampal Slice Data.- 4. Discussion.- 4.1. Insights from the Models of Electrotonic Structure.- 4.2. Models of Cell Circuitry.- 4.3. Contributions of the Slice Preparation to Model Studies.- 4.4. Experimental Directions and Testable Predictions.- 5. References.- 3. Biophysics and Microphysiology of Synaptic Transmission in Hippocampus.- 1. Motivation for Studying the Biophysics and Microphysiology of Cortical Synapses.- 2. Criteria for Selecting a Suitable Cortical Synaptic Preparation.- 2.1. Identifiable Neurons and Synapses.- 2.2. Stable Intracellular Recordings.- 2.3. Minimal Diffusional Barriers.- 2.4. Monosynaptic Connection from Stimulus Site.- 2.5. Minimal Electrotonic Distance between Subsynaptic Membrane and Recording Site.- 2.6. Measurement of Single Quantal Events.- 2.7. Application of Voltage-Clamp Techniques.- 2.8. A Synapse That Satisfies These Criteria in the Hippocampal Slice Preparation.- 3. Development of Voltage-Clamp Techniques for Application to Hippocampal Synapses.- 3.1. Techniques for Voltage Clamping Small Cortical Neurons.- 3.2. The Problem of Space Clamp.- 4. Current- and Voltage-Clamp Studies of Evoked Synaptic Events in Hippocampal Neurons.- 4.1. Analysis of Mossy-Fiber Evoked Synaptic Potentials.- 4.2. Analysis of Mossy-Fiber Evoked Synaptic Currents.- 5. Current- and Voltage-Clamp Studies of Spontaneous Miniature Synaptic Events in Hippocampal Neurons.- 5.1. The Quantum Hypotheses.- 5.2. Discovery of Spontaneous Miniature Synaptic Potentials in Hippocampal Neurons.- 5.3. Current- and Voltage-Clamp Studies of Single Quantal Events.- 6. Implications for Performing a Quantal Analysis of Evoked Release.- 6.1. The Problem of Mixed Synaptic Responses.- 6.2. Some Advantages of Voltage-Clamp Analysis.- 6.3. The Importance of a Suitable Signal-to-Noise Ratio.- 6.4. A Caveat Concerning Release and Quantal Size Statistics.- 7. Significance for Selected Problems in Cortical Physiology.- 7.1. Mechanism of Long-Term Synaptic Potentiation.- 7.2. Role of Dendrites and Their Spines in Synaptic Information Transfer and Integration.- 7.3. Currents Underlying Epileptiform Discharges.- 7.4. Summary and Conclusions.- 8. References.- 4. Hippocampus: Synaptic Pharmacology.- 1. Introduction.- 2. Localization of Transmitters and Endogenous Neuroactive Agents in the Hippocampal Formation.- 2.1. Transmitter Candidates.- 2.2. Neuroactive Peptides.- 3. Cellular Actions of Neuroactive Drugs in Hippocampal Slices.- 3.1. GABA.- 3.2. Acetylcholine.- 3.3. Opioid Peptides.- 3.4. Excitatory Amino Acids.- 4. Conclusions and Future Directions.- 5. References.- 5. Energy Metabolism and Brain Slice Function.- 1. Introduction.- 2. Integrity of the Slice Preparation.- 2.1. Comparison of Energy-Related Parameters in Slices and in Situ.- 2.2. Possible Bases for Compromised Function in the Brain Slice.- 3. Mechanism of Anoxic Damage.- 3.1. Metabolic Changes during Anoxia.- 3.2. Effects of Metabolic Changes Occurring during Anoxia on Neurotransmission.- 3.3. Experimental Evidence Concerning the Mechanism of Synaptic Transmission Failure during Compromised Oxidative Phosphorylation.- 4. References.- 6. Hippocampus: Electrophysiological Studies of Epileptiform Activity in Vitro.- 1. Introduction.- 1.1. Experimental Questions.- 1.2. Terminology.- 2. What Enables Some Cells to Fire Bursts Readily?.- 2.1. Paroxysmal Depolarization Shift (PDS).- 2.2. Regenerative Components of the Burst.- 3. Why Do Cells That Can Fire Burst Potentials Not Do So All the Time?.- 3.1. Inhibitory Postsynaptic Potentials (IPSPs) Prevent Bursts.- 3.2. Feedforward Dendritic Inhibition.- 4. How Does Synchronization of Firing within a Population of Cells Occur?.- 4.1. Imposed Synchrony.- 4.2. “Spontaneous” Synchrony.- 4.3. Electrotonic Interactions.- 5. What Triggers the Switch from Interictal Spiking to Seizures?.- 5.1. Burst Afterhyperpolarizations (AHP).- 5.2. The Late Hyper polarizing Potential (LHP) is a Slow IPSP.- 5.3. Seizure Development.- 6. How Can a Seizure Spread from Epileptic Tissue across Normal Tissue?.- 6.1. Endogenous Opiates.- 6.2. Acetylcholine.- 6.3. Use-Dependent IPSP Depression.- 6.4. Ammonia.- 7. Conclusions.- 8. References.- 7. Correlated Electrophysiological and Biochemical Studies of Hippocampal Slices.- 1. Introduction.- 2. Modification of Stimulation Procedures and Slice Techniques for Biochemical Experiments.- 3. Hippocampal Long-Term Potentiation.- 4. Influences of High-Frequency Stimulation on 3H-Glutamate Binding to Synaptic Membranes.- 4.1. Characteristics of 3H-Glutamate Binding to Hippocampal Synaptic Membranes.- 4.2. Effect of High-Frequency Stimulation on 3H-Glutamate Binding.- 4.3. Calcium Regulation of 3H-Glutamate Binding to Hippocampal Membranes.- 5. High-Frequency Stimulation and Protein Phosphorylation.- 6. Summary.- 7. References.- 8. Optical Monitoring of Electrical Activity: Detection of Spatiotemporal Patterns of Activity in Hippocampal Slices by Voltage-Sensitive Probes.- 1. Introduction.- 1.1. Preview.- 1.2. The Limitations of Current Intracellular Electrical Recording Techniques.- 1.3. The Principle of the Optical Recording Technique.- 2. Optical Monitoring of Changes in Membrane Potential.- 2.1. The Apparatus.- 2.2. Design and Synthesis of Improved Optical Probes.- 2.3. Example of Application to the Study of Single Cells and the Study of Invertebrate CNS.- 3. Optical Recording from Brain Slices.- 3.1. Preparation of the Slices for Optical Recordings.- 3.2. The Correction Procedure for Light-Scattering Signals.- 3.3. Optical Signals from a Stained Slice.- 3.4. Light-Scattering Signals from Unstained Slices.- 3.5. Ca2+ Dependency of the Fast and Slow Responses.- 3.6. Effects of Tetrodotoxin on the Fast Responses.- 3.7. Optical “Artifacts” near the Stimulating Electrode.- 3.8. Properties of the Unmyelinated Axons in the Hippocampus Slice.- 3.9. Postsynaptic Responses.- 3.10. The Cellular Discharges.- 3.11. Examples of Pharmacological Studies: The Effect of Picrotoxin.- 3.12. Visualization of the Spread of Activity in Slices.- 3.13. Comparison between Field Potentials and Optical Recordings.- 3.14. Limitations and Advantages.- 3.15. Future Prospects.- 4. References.- 9. Probing the Extracellular Space of Brain Slices with Ion-Selective Microelectrodes.- 1. Introduction.- 2. The Brain Cell Microenvironment.- 3. Extracellular Ion Changes Produced by Simultaneous Activity of Ensembles of Neurons.- 4. Extracellular Ion Changes Evoked by Individual Cells.- 4.1. Spontaneous Purkinje Cell Activity.- 4.2. Changes in [K+]0 during Simultaneous Intracellular Recording and Current Passage.- 4.3. Changes in [K+]0 Recorded outside Glia Cells during Intracellular Current Passage.- 5. Prospects and Problems.- 6. References.- 10. Electrophysiological Study of the Neostriatum in Brain Slice Preparation.- 1. Introduction.- 2. Methods.- 2.1. Electrode.- 2.2. Slice Chamber.- 2.3. Slice Preparation.- 3. Results and Discussion.- 3.1. Field Potentials.- 3.2. Intracellular Recording.- 3.3. Discussion and Summary.- 4. References.- 11. Locus Coeruleus Neurons.- 1. Introduction.- 2. Methods.- 3. Results.- 3.1. Guinea Pig Locus Coeruleus.- 3.2. Guinea Pig Mesencephalic Nucleus of the Trigeminal Nerve.- 3.3. Rat Locus Coeruleus.- 3.4. Pharmacological Studies.- 4. Discussion.- 5. References.- 12. Neocortex: Cellular Properties and Intrinsic Circuitry.- 1. Introduction.- 2. Early Use of Neocortical Slices.- 3. Notes on Neocortical Slice Methodology.- 4. Properties of Neocortical Slices.- 4.1. Electrophysiology of Single Cells.- 4.2. Synaptic Behavior of Neocortical Neurons.- 4.3. Intrinsic Connectivity of Neocortical Slices.- 5. Conclusions.- 6. References.- 13. Hypothalamic Neurobiology.- 1. Introduction.- 2. The Development of the Hypothalamic Slice Preparation.- 2.1. Motivating Factors.- 2.2. Early Problems Encountered.- 3. Hypothalamic Slice Preparations and Their Uses.- 3.1. Magnocellular Areas.- 3.2. Parvocellular Areas.- 4. Summary and Conclusions.- 5. References.- 14. Brain Slice Work: Some Prospects.- 1. Introduction.- 2. Optimal Conditions.- 3. Slices from New Regions.- 4. New Uses of Slices.- 5. New Approaches in Slice Experiments.- 6. The Need for Correlation.- 7. References.- Appendix: Brain Slice Methods.- 1. Introduction.- 2. Preparation of Slices.- 2.1. Slicing the Brain.- 2.2. Slice Chambers.- 2.3. Bathing Medium Composition.- 2.4. Slice Thickness.- 3. Evaluation of Slice Data.- 3.1. General Remarks.- 3.2. Electrophysiology.- 3.3. Histology.- 3.4. Metabolism.- 3.5. Spreading Depression.- 4. Methods of Drug Application.- 4.1. Superfusion.- 4.2. TheNanodrop.- 4.3. Iontophoresis.- 4.4. The Pressure Pipette.- 4.5. Summary.- 5. Concluding Statement.- 6. References.
Date de parution : 06-2012
Ouvrage de 442 p.
15.2x22.9 cm
Disponible chez l'éditeur (délai d'approvisionnement : 15 jours).
Prix indicatif 105,49 €
Ajouter au panierThème de Brain Slices :
Mots-clés :
biology; brain; cortex; depression; document; experiment; information; myelin; neurobiology; neurons; physiology; research
© 2024 LAVOISIER S.A.S.