Biochemistry An Integrative Approach
Auteur : Tansey John T.
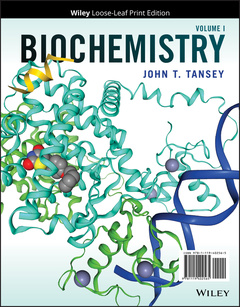
Biochemistry: An Integrative Approach is addressed to premed, biochemistry, and life science majors taking a one-semester biochemistry course. This version includes the first 12 chapters and should only be used for one-semester biochemistry courses.
Biochemistry addresses the diverse needs of premed, biochemistry, and life science majors by presenting relevant material while still preserving a chemical perspective. Presented within the next generation of WileyPLUS,Biochemistry emphasizes worked problems through video walkthroughs, interactive elements and expanded end-of-chapter problems with a wide range of subject matter and difficulty. The worked problems in the course are both qualitative and quantitative and model for students the biochemical reasoning they need to practice. Students will often be asked to analyze data and make critical assessments of experiments.
1 The Chemical Foundations of Biochemistry 1
Chemistry in Context 1
1.1 General Chemical Principles 2
1.1.1 The basic principles of thermodynamics describe all systems 2
1.1.2 Equilibrium describes a specific thermodynamic state 7
1.1.3 Kinetics describes the rate of chemical reactions 8
1.1.4 Thermodynamics, equilibrium, and kinetics are used together to describe biochemical reactions and systems 9
1.2 Fundamental Concepts of Organic Chemistry 11
1.2.1 Chemical properties and reactions can be sorted by functional groups 11
1.2.2 The solubility and polarity of a molecule can be determined from its structure 12
1.2.3 Reaction mechanisms attempt to explain how a reaction occurs 13
1.2.4 Many biochemical molecules are polymers 14
1.3 The Chemistry of Water 15
1.3.1 The structure of water provides clues about its properties 16
1.3.2 Hydrogen bonding among water molecules is one of the most important weak forces in biochemistry 17
1.3.3 Water can ionize to acids and bases in biochemical systems 18
2 Nucleic Acids 26
Nucleic Acids in Context 26
2.1 Nucleic Acids Have Distinct Structures 27
2.1.1 Nucleic acids can be understood at the chemical level 28
2.1.2 The complex shapes of nucleic acids are the result of numerous weak forces 30
2.2 Nucleic Acids Have Many Cellular Functions 37
2.2.1 Replication is the process by which cells copy DNA 37
2.2.2 Transcription is the copying of DNA into an RNA message 40
2.2.3 Translation is the synthesis of proteins by ribosomes using an mRNA code 41
2.2.4 Regulation of replication, transcription, and translation is critical to an organism’s survival and propagation 42
2.2.5 Viruses and retroviruses use the cell’s own machinery to reproduce 43
2.3 The Manipulation of Nucleic Acids Has Transformed Biochemistry 45
2.3.1 DNA can be easily manipulated and analyzed in vitro 45
2.3.2 DNA can be used to drive protein expression 53
2.3.3 Techniques can be used to silence genes in organisms 59
3 Proteins I: An Introduction to Protein Structure and Function 67
Proteins in Context 67
3.1 Amino Acid Chemistry 69
3.1.1 The structure of amino acids dictates their chemical properties 69
3.1.2 The side chains of amino acids impart unique properties 70
3.1.3 Amino acids have various roles in biochemistry 72
3.2 Proteins Are Polymers of Amino Acids 74
3.2.1 Both peptides and proteins are polymers of amino acids 74
3.2.2 The peptide bond has special characteristics 76
3.2.3 Many proteins require inorganic ions or small organic molecules to function 76
3.2.4 Amino acids can be modified within proteins 77
3.3 Proteins Are Molecules of Defined Shape and Structure 79
3.3.1 The primary structure of a protein is its amino acid sequence 79
3.3.2 The secondary structure of a protein is comprised of a few conserved structures 81
3.3.3 The tertiary structure of a protein is the organization of secondary structures into conserved motifs 84
3.3.4 The quaternary structure of a protein describes how individual subunits interact 88
3.4 Examples of Protein Structures and Functions 89
3.4.1 Aquaporin, a transmembrane pore 90
3.4.2 Chymotrypsin, an enzyme 90
3.4.3 Collagen, a structural protein 91
3.4.4 Hemoglobin, a transport protein 92
3.4.5 Immunoglobulins, binding proteins 92
3.4.6 Insulin, a signaling protein 93
3.4.7 Myosin, a molecular motor 94
4 Proteins II: Enzymes 101
Enzymes in Context 101
4.1 Regarding Enzymes 103
4.1.1 Enzymes are protein catalysts 103
4.1.2 Enzymatically catalyzed reactions can be categorized 105
4.1.3 How do enzymes work? 106
4.2 Enzymes Increase Reaction Rate 108
4.2.1 A review of chemical rates 108
4.2.2 The Michaelis-Menten equation relates enzymatic rates to measurable parameters 109
4.2.3 The Michaelis constant has several meanings 111
4.2.4 Kinetic data can be graphically analyzed 112
4.2.5 kcat/KM is a measure of catalytic efficiency 112
4.2.6 Some enzymes approach catalytic perfection 112
4.2.7 Enzymatic reactions may be inhibited through one of several different mechanisms 113
4.2.8 Many reactions have more than one substrate 116
4.3 The Mechanism of an Enzyme Can Be Deduced from Structural, Kinetic, and Spectral Data 117
4.3.1 Enzymatically catalyzed reactions have common properties 118
4.3.2 Examining examples of enzymatic reaction mechanisms illustrates underpinning principles 119
4.3.3 Mechanisms are elucidated using a combination of experimental techniques 125
4.4 Examples of Enzyme Regulation 127
4.4.1 Covalent modifications are a common means of enzyme regulation 127
4.4.2 Allosteric regulators bind at sites other than the active site 129
5 Membranes and an Introduction to Signal Transduction 138
Biochemistry in Context 138
5.1 Membrane Structure and Function 139
5.1.1 The chemical properties of the membrane components dictate the characteristics of the membrane 140
5.1.2 Other aspects of membrane structure 145
5.1.3 Membrane fusion and membrane budding 146
5.2 Signal Transduction 149
5.2.1 General principles underlie signal transduction 149
5.2.2 The protein kinase A (PKA) signaling pathway is activated by cyclic AMP 150
5.2.3 Insulin is an important metabolic regulator and growth factor 153
5.2.4 The AMP kinase (AMPK) signaling pathway coordinates metabolic pathways in the cell and in the body 154
6 Carbohydrates I: Mono- and Disaccharides, Glycolysis, Gluconeogenesis, and the Fates of Pyruvate 161
Carbohydrates in Context 161
6.1 Properties, Nomenclature, and Biological Functions of Monosaccharides 163
6.1.1 Monosaccharides are the simplest carbohydrates 163
6.1.2 Monosaccharides form hemiacetals and hemiketals 165
6.1.3 Monosaccharides form heterocyclic structures 165
6.1.4 Monosaccharides can be chemically modified 167
6.1.5 Carbohydrates can be classified as reducing sugars or nonreducing sugars 168
6.2 Properties, Nomenclature, and Biological Functions of Complex Carbohydrates 170
6.2.1 Common disaccharides include lactose, sucrose, and maltose 170
6.2.2 Trisaccharides and oligosaccharides contain three or more monosaccharide units bound by glycosidic linkages 173
6.2.3 Common polysaccharides function to store energy or provide structure 174
6.3 Glycolysis and an Introduction to Metabolic Pathways 177
6.3.1 Metabolic pathways describe how molecules are built up or broken down 178
6.3.2 Glycolysis is the process by which glucose is broken into pyruvate 180
6.4 Gluconeogenesis 193
6.4.1 Gluconeogenesis differs from glycolysis at four reactions 193
6.4.2 The regulation of gluconeogenesis takes place at several different levels 195
6.5 The Fates of Pyruvate 196
6.5.1 Pyruvate can be decarboxylated to acetyl-CoA by pyruvate dehydrogenase 197
6.5.2 Pyruvate can be converted to lactate by lactate dehydrogenase 200
6.5.3 Pyruvate can be transaminated to alanine 201
6.5.4 Pyruvate can be carboxylated to oxaloacetate by pyruvate carboxylase 201
6.5.5 Microbes can decarboxylate pyruvate into acetaldehyde 201
7 The Common Catabolic Pathway: Citric Acid Cycle, the Electron Transport Chain, and ATP Biosynthesis 209
Electron Transport in Context 209
7.1 The Citric Acid Cycle 211
7.1.1 There are eight reactions in the citric acid cycle 212
7.1.2 The citric acid cycle is regulated at multiple places and by several different mechanisms 218
7.1.3 Anaplerotic reactions of the citric acid cycle replenish intermediates 219
7.2 The Electron Transport Chain 223
7.2.1 Electron transport occurs through a series of redox active centers from higher to lower potential energy 224
7.2.2 Complex I (NADH dehydrogenase) transfers electrons from NADH to ubiquinone via a series of iron-sulfur centers 229
7.2.3 Complex II is the citric acid cycle enzyme succinate dehydrogenase 231
7.2.4 Complex III is ubiquinone/cytochrome c reductase 233
7.2.5 Cytochrome c is a soluble electron carrier 235
7.2.6 In complex IV, oxygen is the terminal electron carrier 235
7.2.7 The entire complex working as one: the respirasome 238
7.3 ATP Biosynthesis 241
7.3.1 The structure of ATP synthase underlies its function 241
7.3.2 ATP synthase acts as a molecular machine driving the assembly of ATP molecules 243
7.3.3 Other ATPases serve as ion pumps 245
7.3.4 Inhibitors of the ATPases can be powerful drugs or poisons 246
8 Carbohydrates II: Glycogen Metabolism, the Pentose Phosphate Pathway, Glycoconjugates, and Extracellular Matrices 253
Polysaccharides in Context 253
8.1 Glycogen Metabolism 255
8.1.1 Glycogenesis is glycogen biosynthesis 256
8.1.2 Glycogenolysis is glycogen breakdown 257
8.1.3 The regulation of glycogenesis and glycogenolysis 259
8.2 The Pentose Phosphate Pathway 264
8.2.1 The oxidative phase of the pentose phosphate pathway produces NADPH and ribulose-5- phosphate 264
8.2.2 The nonoxidative phase of the pentose phosphate pathway results in rearrangement of monosaccharides 266
8.2.3 Regulation of the pentose phosphate pathway 267
8.2.4 The pentose phosphate pathway in health and disease 268
8.2.5 Xylulose-5-phosphate is a master regulator of carbohydrate and lipid metabolism 271
8.3 Carbohydrates in Glycoconjugates 273
8.3.1 Glycoproteins 273
8.3.2 Glycolipids 276
8.3.3 Proteoglycans and non-proteoglycan polysaccharides 279
8.3.4 Peptidoglycans 282
8.4 Extracellular Matrices and Biofilms 284
8.4.1 Eukaryotic extracellular matrix proteins 284
8.4.2 Biofilms are composed of microbes living in a secreted matrix 290
9 Lipids I: Fatty Acids, Steroids, and Eicosanoids; Beta-Oxidation and Fatty Acid Biosynthesis 300
Lipids in Context 300
9.1 Properties, Nomenclature, and Biological Functions of Lipid Molecules 302
9.1.1 Fatty acids are a common building block of many lipids 302
9.1.2 Neutral lipids are storage forms of fatty acids or cholesterol 305
9.1.3 Phospholipids are important in membrane formation 306
9.1.4 All steroids and bile salts are derived from cholesterol 309
9.1.5 Eicosanoids are potent signaling molecules derived from 20-carbon polyunsaturated fatty acids 310
9.2 Fatty Acid Catabolism 311
9.2.1 Fatty acids must be transported into the mitochondrial matrix before catabolism can proceed 312
9.2.2 Fatty acids are oxidized to acetyl-CoA by β-oxidation 312
9.2.3 Beta-oxidation is regulated at two different levels 316
9.3 Fatty Acid Biosynthesis 317
9.3.1 Two major enzyme complexes are involved in fatty acid biosynthesis 318
9.3.2 Elongases and desaturases in the endoplasmic reticulum increase fatty acid diversity 326
9.3.3 The formation of malonyl-CoA by acetyl-CoA carboxylase is the regulated and rate-determining step of fatty acid biosynthesis 327
9.4 Ketone Body Metabolism 329
9.4.1 Ketone bodies are made from acetyl-CoA 330
9.4.2 Ketone bodies can be thought of as a water-soluble fuel source used in the absence of carbohydrates 330
9.5 Steroid Metabolism 332
9.5.1 Cholesterol is synthesized in the liver through the addition of energetically activated isoprene units 332
9.5.2 Steroid hormones are derived from cholesterol 336
9.5.3 Bile salts are steroid detergents used in the digestion of fats 337
9.6 Eicosanoid and Endocannabinoid Metabolism 339
9.6.1 Eicosanoids are classified by the enzymes involved in their synthesis 339
9.6.2 Endocannabinoids such as anandamide are also arachidonate derivatives 341
10 Lipids II: Metabolism and Transport of Complex Lipids 351
Complex Lipids in Context 351
10.1 Phospholipid Metabolism 353
10.1.1 Glycerophospholipids are derived from phosphatidate or diacylglycerol using activated carriers 354
10.1.2 Sphingolipids are synthesized from ceramide 355
10.1.3 Phospholipases and sphingolipases cleave at specific sites 358
10.2 Digestion of Triacylglycerols 361
10.2.1 Triacylglycerol digestion begins in the gastrointestinal tract 361
10.2.2 Dietary lipids are absorbed in the small intestine and pass into lymph before entering the circulation 363
10.2.3 Several molecules affect neutral lipid digestion 365
10.3 Transport of Lipids in the Circulation 367
10.3.1 Lipoproteins have a defined structure and composition, and transport lipids in the circulation 367
10.3.2 The trafficking of lipoproteins in the blood can be separated conceptually into three different paths 369
10.3.3 Brain lipids are transported on apo E-coated discs 372
10.3.4 Fatty acids and hydrophobic hormones are transported by binding to carrier proteins 374
10.4 Entry of Lipids into the Cell 377
10.4.1 Fatty acids can enter the cell via diffusion or by protein-mediated transport 377
10.4.2 Lipoprotein particles and many other complexes enter the cell via receptor-mediated endocytosis 377
10.5 Neutral Lipid Biosynthesis 380
10.5.1 Triacylglycerols are synthesized by different pathways depending on the tissue 380
10.5.2 Triacylglycerol metabolism and phosphatidate metabolism are enzymatically linked 382
10.6 Lipid Storage Droplets, Fat Storage, and Mobilization 384
10.6.1 Bulk neutral lipids in the cell are stored in a specific organelle, the lipid storage droplet 384
10.6.2 Specific phosphorylation of lipases and lipid droplet proteins regulate lipolysis (triacylglycerol breakdown) 384
10.6.3 Triacylglycerol metabolism is regulated at several levels 387
10.7 Lipid Rafts as a Biochemical Entity 388
10.7.1 Lipid rafts are loosely associated groups of sphingolipids and cholesterol found in the plasma membrane 388
10.7.2 Lipid rafts have been broadly grouped into two categories: caveolae and non-caveolar rafts 388
11 Amino Acid and Amine Metabolism 397
Amine Metabolism in Context 397
11.1 Digestion of Proteins 399
11.1.1 Protein digestion begins in the stomach 399
11.1.2 Protein digestion continues in the small intestine, aided by proteases 401
11.1.3 Amino acids are absorbed in the small intestine 401
11.1.4 Amino acids serve many biological roles in the organism 403
11.2 Transamination and Oxidative Deamination 404
11.2.1 Ammonia can be removed from an amino acid in two different ways 405
11.2.2 The glucose–alanine shuttle moves nitrogen to the liver and delivers glucose to tissues that need it 405
11.2.3 Glutamine is also important in nitrogen transport 406
11.3 The Urea Cycle 409
11.3.1 Ammonia detoxification begins with the synthesis of carbamoyl phosphate 409
11.3.2 The urea cycle synthesizes urea and other metabolic intermediates 409
11.3.3 Nitrogen metabolism is regulated at different levels 412
11.3.4 Mechanisms for elimination of nitrogenous wastes differ between mammals and non-mammals 412
11.3.5 Some mammals have adapted to high- or low-protein diets 413
11.4 Pathways of Amino Acid Carbon Skeleton Scavenging 414
11.4.1 Three-carbon skeletons produce pyruvate 416
11.4.2 Four-carbon skeletons produce oxaloacetate 417
11.4.3 Five-carbon skeletons produce α-ketoglutarate 418
11.4.4 Methionine, valine, and isoleucine produce succinyl-CoA 418
11.4.5 Other amino acids produce acetyl-CoA, acetoacetate, or fumarate 418
11.5 The Detoxification of Other Amines and Xenobiotics 423
11.5.1 Phase I metabolism makes molecules more hydrophilic through oxidative modification 424
11.5.2 Phase II metabolism couples molecules to bulky hydrophilic groups 427
11.6 The Biochemistry of Renal Function 429
11.6.1 Molecules smaller than proteins are filtered out of the blood by the glomerulus 430
11.6.2 Water, glucose, and electrolytes are reabsorbed in the proximal convoluted tubule, loop of Henle, and distal convoluted tubule 431
12 Regulation and Integration of Metabolism 440
Metabolism in Context 440
12.1 A Review of the Pathways and Crossroads of Metabolism 442
12.1.1 The pathways of metabolism are interconnected 442
12.1.2 Several metabolites are at the intersection of multiple pathways 443
12.2 Organ Specialization and Metabolic States 446
12.2.1 Different organs play distinct roles in metabolism 447
12.2.2 The organism shifts between different metabolic states depending on access to food 450
12.3 Communication between Organs 455
12.3.1 Organs communicate using hormones 455
12.3.2 Hormonal signals to the brain regulate appetite and metabolism 458
12.3.3 Transcription factors and histone acetylases and deacetylases regulate metabolism in the longer term 460
12.4 Metabolic Disease 463
12.4.1 Diseases of excess: obesity, diabetes, and metabolic syndrome 463
12.4.2 Diseases of absence: starvation, cachexia, and cancer 467
12.4.3 Diseases of indulgence: alcohol overconsumption 468
12.4.4 Other metabolic states 469
Techniques 477
solutions 493
Glossary 509
Index I-1
John T. Tansey received his B.S. in Biochemistry and Molecular Biology from the University of Massachusetts, Amherst, and his Ph.D. in Biochemistry from Wake Forest University. Following his graduate degree, John undertook a postdoctoral fellowship at the National Institutes of Health in the lab of Dr. Constantine Londos where he led the team that generated and characterized the perilipin 1 knockout mouse. John's scientific interests are a natural extension of his postdoctoral studies and focus on the structure and function of the perilipins, a class of lipid droplet proteins that regulate neutral lipid storage and breakdown. John has been teaching for the past seventeen years in the Chemistry department and Biochemistry and Molecular Biology program at Otterbein University, a primarily undergraduate institution outside Columbus, Ohio. He has also taught at the community college and graduate level. John was awarded both the Best New Teacher and Master Teacher awards from his university. He is an education fellow of the American Society for Biochemistry and Molecular Biology (ASBMB) and is a member of the editorial board of Biochemistry and Molecular Biology Education (BAMBEd). In addition to his basic science interests, he has studied the use of science fiction or other readings to promote engagement and discussion in science classes, integration of codes of conduct and basic ethics in science courses, and development of curricula and tools for programmatic assessment. When he isn't teaching or in the lab, John enjoys cooking, the outdoors, games, and a good laugh.
Date de parution : 04-2019
Ouvrage de 560 p.
21.3x27.7 cm