Thermomechanical Fatigue of Ceramic-Matrix Composites
Auteur : Li Longbiao
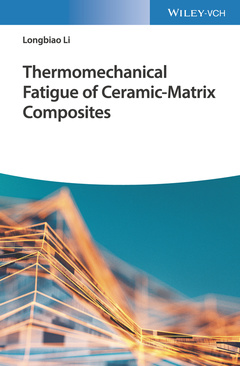
The book systematically introduces the thermomechanical fatigue behavior of fiber-reinforced ceramic-matrix composites (CMCs) and environmental barrier coatings, including cyclic loading/unloading tensile behavior, cyclic fatigue behavior, dwell-fatigue behavior, thermomechanical fatigue behavior, and interface degradation behavior. It discusses experimental verification of CMCs and explains how to determine the thermomechanical properties. It also presents damage evolution models, lifetime prediction methods, and interface degradation rules.
Thermomechanical Fatigue of Ceramic-Matrix Composites offers chapters covering unidirectional ceramic-matrix composites and cross-ply and 2D woven ceramic-matrix composites. For cyclic fatigue behavior of CMCs, it looks at the effects of fiber volume fraction, fatigue peak stress, fatigue stress ratio, matrix crack spacing, matrix crack mode, and woven structure on fatigue damage evolution. Both the Dwell-fatigue damage evolution and lifetime predictions models are introduced in the next chapter. Experimental comparisons of the cross-ply SiC/MAS composite, 2D SiC/SiC composite, and 2D NextelTM 720/Alumina composite are also included. Remaining sections examine: thermomechanical fatigue hysteresis loops; in-phase thermomechanical fatigue damage; out-of-phase thermomechanical fatigue; interface degradation models; and much more.
-Offers unique content dedicated to thermomechanical fatigue behavior of ceramic-matrix composites (CMCs) and environmental barrier coatings
-Features comprehensive data tables and experimental verifications
-Covers a highly application-oriented subject?CMCs are being increasingly utilized in jet engines, industrial turbines, and exhaust systems
Thermomechanical Fatigue of Ceramic-Matrix Composites is an excellent book for developers and users of CMCs, as well as organizations involved in evaluation and characterization of CMCs. It will appeal to materials scientists, construction engineers, process engineers, and mechanical engineers.
1 Cyclic Loading/Unloading Tensile Fatigue of Ceramic-Matrix Composites 1
1.1 Introduction 1
1.2 Unidirectional Ceramic-Matrix Composites 2
1.2.1 Materials and Experimental Procedures 3
1.2.1.1 C/SiC Composite 3
1.2.1.2 C/Si3N4 and SiC/Si3N4 Composites 3
1.2.1.3 SiC/CAS Composite 4
1.2.2 Theoretical Analysis 4
1.2.2.1 Stress Analysis 4
1.2.2.2 Matrix Cracking 6
1.2.2.3 Interface Debonding 7
1.2.2.4 Fiber Failure 8
1.2.2.5 Hysteresis Theories 9
1.2.3 Results and Discussion 13
1.2.3.1 Effect of Fiber Volume Fraction on Fatigue Hysteresis Loops and Fatigue Hysteresis-Based Damage Parameters 13
1.2.3.2 Effect of Matrix Cracking Density on Fatigue Hysteresis Loops and Fatigue Hysteresis-Based Damage Parameters 15
1.2.3.3 Effect of Fiber/Matrix Interface Shear Stress on Fatigue Hysteresis Loops and Fatigue Hysteresis-Based Damage Parameters 16
1.2.3.4 Effect of Fiber/Matrix Interface Debonded Energy on Fatigue Hysteresis Loops and Fatigue Hysteresis-Based Damage Parameters 20
1.2.3.5 Effect of Fiber Failure on Fatigue Hysteresis Loops and Fatigue Hysteresis-Based Damage Parameters 22
1.2.4 Experimental Comparisons 24
1.2.4.1 C/SiC Composite 24
1.2.4.2 C/Si3N4 Composite 30
1.2.4.3 SiC/Si3N4 Composite 33
1.2.4.4 SiC/CAS Composite 38
1.3 Cross-Ply and 2D Woven Ceramic-Matrix Composites 43
1.3.1 Materials and Experimental Procedures 47
1.3.1.1 C/SiC Composite 47
1.3.1.2 SiC/SiC Composite 48
1.3.2 Theoretical Analysis 49
1.3.2.1 Stress Analysis 49
1.3.2.2 Transverse and Matrix Cracking 58
1.3.2.3 Interface Debonding 60
1.3.2.4 Hysteresis Theories 62
1.3.3 Results and Discussions 75
1.3.3.1 Effect of Fiber Volume Fraction on the Interface Sliding and Fatigue Hysteresis Loops 75
1.3.3.2 Effect of Fatigue Peak Stress on the Interface Sliding and Fatigue Hysteresis Loops 77
1.3.3.3 Effect of Matrix Crack Spacing on the Interface Sliding and Fatigue Hysteresis Loops 79
1.3.3.4 Effect of Interface Properties on the Interface Sliding and Fatigue Hysteresis Loops 81
1.3.3.5 Effect of Matrix Racking Mode Proportion on Interface Sliding and Fatigue Hysteresis Loops 85
1.3.4 Experimental Comparisons 87
1.3.4.1 Cross-Ply C/SiC Composite 87
1.3.4.2 2D SiC/SiC Composite 94
1.4 2.5D and 3D Ceramic-Matrix Composites 103
1.4.1 Materials and Experimental Procedures 104
1.4.1.1 2.5D C/SiC Composite 104
1.4.1.2 3D Braided C/SiC Composite 104
1.4.1.3 3D Needled C/SiC Composite 105
1.4.2 HysteresisTheories 105
1.4.2.1 Interface Slip Case 1 105
1.4.2.2 Interface Slip Case 2 106
1.4.2.3 Interface Slip Case 3 107
1.4.2.4 Hysteresis Loops 107
1.4.3 Experimental Comparisons 108
1.4.3.1 2.5D C/SiC Composite 108
1.4.3.2 3D Braided C/SiC Composite 110
1.4.3.3 3D Needled C/SiC Composite 112
1.5 Conclusions 112
References 112
2 Cyclic Fatigue Behaviors of Ceramic-Matrix Composites 117
2.1 Introduction 117
2.2 Materials and Experimental Procedures 117
2.2.1 Unidirectional C/SiC Composite 117
2.2.2 Cross-Ply C/SiC Composite 118
2.2.3 2D SiC/SiC Composite at 1000°C 119
2.2.4 2D SiC/SiC Composite at 1200°C 120
2.2.5 2D SiC/SiC Composite at 1300°C 120
2.2.6 3D SiC/SiC Composite at 1300°C 121
2.3 Hysteresis-Based Damage Parameters 121
2.4 Results and Discussions 122
2.4.1 Effects of Fiber Volume Fraction on Fatigue Damage Evolution 123
2.4.2 Effects of Fatigue Peak Stress on Fatigue Damage Evolution 125
2.4.3 Effects of Fatigue Stress Ratio on Fatigue Damage Evolution 127
2.4.4 Effects of Matrix Crack Spacing on Fatigue Damage Evolution 128
2.4.5 Effects of Matrix Crack Mode on Fatigue Damage Evolution 129
2.4.6 Effects of Woven Structure on Fatigue Damage Evolution 133
2.5 Experimental Comparisons 135
2.5.1 Unidirectional CMCs 135
2.5.1.1 SiC/CAS Composite at Room Temperature 135
2.5.1.2 SiC/CAS-II Composite at Room Temperature 137
2.5.1.3 SiC/1723 Composite at Room Temperature 140
2.5.1.4 C/SiC Composite at Room Temperature 143
2.5.1.5 C/SiC Composite at Elevated Temperature 147
2.5.2 Cross-Ply CMCs 152
2.5.2.1 SiC/CAS Composite at Room Temperature 152
2.5.2.2 C/SiC Composite at Room Temperature 155
2.5.2.3 C/SiC Composite at 800°C in Air Atmosphere 156
2.5.2.4 SiC/MAS-L Composite at 800 and 1000°C in Inert Atmosphere 158
2.5.3 2D CMCs 158
2.5.3.1 SiC/SiC Composite at 600, 800, and 1000°C in Inert Atmosphere 158
2.5.3.2 SiC/SiC Composite at 1000∘C in Air and in Steam Atmospheres 164
2.5.3.3 SiC/SiC Composite at 1200∘C in Air and in Steam Atmospheres 191
2.5.3.4 SiC/SiC Composite at 1300∘C in Air Atmosphere 209
2.5.4 3D Braided CMCs 226
2.6 Discussions 229
2.6.1 Cyclic Fatigue at Room Temperature 229
2.6.2 Cyclic Fatigue at Elevated Temperature 233
2.6.3 Comparison Analysis 238
2.7 Conclusions 245
References 246
3 Dwell-Fatigue Behavior of Ceramic-Matrix Composites 249
3.1 Introduction 249
3.2 Theoretical Analysis 251
3.2.1 Dwell-Fatigue Damage Evolution Model 253
3.2.2 Dwell-Fatigue Lifetime Prediction Model 256
3.3 Results and Discussions 258
3.3.1 Effects of Hold Time on Dwell Fatigue Damage Evolution 258
3.3.2 Effects of Stress Level on Dwell Fatigue Damage Evolution 263
3.3.3 Effects of Matrix Crack Spacing on Dwell Fatigue Damage Evolution 268
3.3.4 Effects of Fiber Volume Fraction on Dwell Fatigue Damage Evolution 272
3.3.5 Effects of Oxidation Temperature on Dwell Fatigue Damage Evolution 276
3.4 Experimental Comparisons 280
3.4.1 Cross-Ply SiC/MAS Composite 280
3.4.1.1 566∘C in Air Atmosphere 280
3.4.1.2 1093∘C in AirAtmosphere 288
3.4.1.3 Comparison Analysis 296
3.4.2 2D SiC/SiC Composite 301
3.4.3 2D Nextel 720/Alumina Composite 303
3.5 Conclusions 304
References 305
4 Thermomechanical Fatigue Behaviors of Ceramic-Matrix Composites 309
4.1 Introduction 309
4.2 Theoretical Analysis 310
4.2.1 Thermomechanical Stress Analysis 310
4.2.2 Thermomechanical Damage Parameters 312
4.3 Thermomechanical Fatigue Hysteresis Loops 313
4.3.1 Results and Discussions 313
4.3.1.1 Effects of Fiber Volume Fraction on the Thermomechanical Fatigue Hysteresis Loops and Fiber/Matrix Interface Sliding 313
4.3.1.2 Effects of Fatigue Peak Stress on the Thermomechanical Fatigue Hysteresis Loops and Fiber/Matrix Interface Sliding 317
4.3.1.3 Effects of Matrix Crack Spacing on the Thermomechanical Fatigue Hysteresis Loops and Fiber/Matrix Interface Sliding 321
4.3.1.4 Effects of Fiber/Matrix Interface Frictional Coefficient on the Thermomechanical Fatigue Hysteresis Loops and Fiber/Matrix Interface Sliding 325
4.3.1.5 Effects of Interface Debonded Energy on the Thermomechanical Fatigue Hysteresis Loops and Fiber/Matrix Interface Sliding 328
4.3.1.6 Effects of Thermal Cyclic Temperature Range on the Thermomechanical Fatigue Hysteresis Loops and Fiber/Matrix Interface Sliding 332
4.3.2 Experimental Comparisons 336
4.3.2.1 Isothermal Fatigue Hysteresis Loops 336
4.3.2.2 In-Phase Thermomechanical Fatigue Hysteresis Loops 341
4.3.2.3 Out-of-phase Thermomechanical Fatigue Hysteresis Loops 344
4.4 In-phase Thermomechanical Fatigue Damage 345
4.4.1 Results and Discussions 347
4.4.1.1 Effects of Fiber Volume Fraction on In-phase Thermomechanical Fatigue Damage Evolution 348
4.4.1.2 Effects of Fatigue Peak Stress on In-phase Thermomechanical Fatigue Damage Evolution 354
4.4.1.3 Effects of Matrix Stochastic Cracking on In-phase Thermomechanical Fatigue Damage Evolution 357
4.4.1.4 Effects of Interface Properties on In-phase Thermomechanical Fatigue Damage Evolution 361
4.4.1.5 Effects of Thermal Cyclic Temperature Range on In-phase Thermomechanical Fatigue Damage Evolution 365
4.4.1.6 Comparisons Between In-phase Thermomechanical and Isothermal Fatigue Loading 368
4.4.2 Experimental Comparisons 370
4.4.2.1 Thermomechanical Fatigue Loading 371
4.4.2.2 Isothermal Fatigue Loading 372
4.5 Out-of-phase Thermomechanical Fatigue 373
4.5.1 Results and Discussions 374
4.5.1.1 Effects of Fiber Volume Fraction on Out-of-phase Thermomechanical Fatigue Damage Evolution 374
4.5.1.2 Effects of Fatigue Peak Stress on Out-of-phase Thermomechanical Fatigue Damage Evolution 379
4.5.1.3 Effects of Matrix Crack Spacing on Out-of-phase Thermomechanical Fatigue Damage Evolution 383
4.5.1.4 Effects of Interface Frictional Coefficient on Out-of-phase Thermomechanical Fatigue Damage Evolution 386
4.5.1.5 Effects of Thermal Cyclic Temperature Range Out-of-phase Thermomechanical Fatigue Damage Evolution 390
4.5.1.6 Comparisons Between In-phase/Out-of-phase Thermomechanical Fatigue and Isothermal Fatigue Loading 393
4.5.2 Experimental Comparisons 397
4.5.2.1 Out-of-phase Thermomechanical Fatigue Loading at the Temperature Range from 566 to 1093∘C 397
4.5.2.2 Isothermal Fatigue Loading at 566∘C 399
4.5.2.3 Isothermal Fatigue Loading at 1093∘C 401
4.6 Thermomechanical Fatigue with Different Phase Angles 403
4.6.1 Results and Discussions 403
4.6.1.1 Effects of Fiber Volume Fraction on Thermomechanical Fatigue Damage Evolution 408
4.6.1.2 Effects of Fatigue Peak Stress on Thermomechanical Fatigue Damage Evolution 416
4.6.1.3 Effects of Matrix Crack Spacing on Thermomechanical Fatigue Damage Evolution 426
4.6.2 Experimental Comparisons 432
4.6.2.1 In-phase Thermomechanical Fatigue 433
4.6.2.2 Out-of-phase Thermomechanical Fatigue 433
4.7 Conclusions 434
References 434
5 Interface Degradation of Ceramic-Matrix Composites Under Thermomechanical Fatigue Loading 437
5.1 Introduction 437
5.2 Interface Degradation Models 438
5.2.1 Interface Slip Case 1 438
5.2.2 Interface Slip Case 2 439
5.2.3 Interface Slip Case 3 439
5.2.4 Interface Slip Case 4 440
5.2.5 Hysteresis Loops and Hysteresis-Based Damage Parameters 441
5.3 Experimental Comparisons 445
5.3.1 Unidirectional C/SiC Composite 445
5.3.1.1 Room Temperature 445
5.3.1.2 Elevated Temperature 451
5.3.1.3 Comparison Analysis 456
5.3.2 Unidirectional SiC/Si3N4 Composite 457
5.3.2.1 Room Temperature 457
5.3.2.2 Elevated Temperature 461
5.3.2.3 Comparison Analysis 467
5.3.3 2D SiC/SiC Composite 468
5.3.3.1 Room Temperature 468
5.3.3.2 Elevated Temperature 470
5.3.3.3 Comparison Analysis 472
5.4 Conclusions 473
References 474
Index 475
Longbiao Li, PhD,is Lecturer in the College of Civil Aviation at Nanjing University of Aeronautics and Astronautics in China, where he received his PhD. His research focuses on the thermomechanical fatigue of high-temperature ceramic-matrix composites. He has published more than 100 journal articles.
Date de parution : 12-2019
Ouvrage de 496 p.
17.8x25.2 cm