Quantum Mechanics for Chemistry, 1st ed. 2023
Auteur : Jang Seogjoo J.
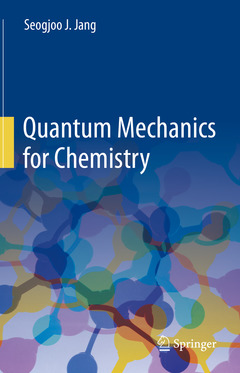
This textbook forms the basis for an advanced undergraduate or graduate level quantum chemistry course, and can also serve as a reference for researchers in physical chemistry and chemical physics. In addition to the standard core topics such as principles of quantum mechanics, vibrational and rotational states, hydrogen-like molecules, perturbation theory, variational principles, and molecular orbital theories, this book also covers essential theories of electronic structure calculation, the primary methods for calculating quantum dynamics, and major spectroscopic techniques for quantum measurement. Plus, topics that are overlooked in conventional textbooks such as path integral formulation, open system quantum dynamics methods, and Green?s function approaches are addressed. This book helps readers grasp the essential quantum mechanical principles and results that serve as the foundation of modern chemistry and become knowledgeable in major methods of computational chemistry and spectroscopic experiments being conducted by present-day researchers. Dirac notation is used throughout, and right balance between comprehensiveness, rigor, and readability is achieved, ensuring that the book remains accessible while providing all the relevant details. Complete with exercises, this book is ideal for a course on quantum chemistry or as a self-study resource.
Chapter 1. Concepts and Assumptions of Quantum Mechanics
1.1 Assumptions of Classical Mechanics
1.2 Concepts of Quantum Mechanics
1.2.1 Quantization of Light Energy and Planck constant
1.2.2 de Broglie’s Theory of Material Wave
1.2.3 Uncertainty Principle
1.2.4 Wave-Particle Duality
1.3 Schrödinger Equation (in one dimension) and Probability Amplitude
1.3.1 Stationary States
1.3.2 Eigenfunction and eigenvalue
1.4 Example of a Quantum Particle in a One-Dimensional Box
1.4.1 Time Independent Schrödinger Equation
1.4.2 Time Dependent States
1.4.3 Completeness
Chapter 2. Dirac Notation for Quantum States and Operators
2.1 Ket, Bra, and Products2.2 Operators
2.2.1 Hermitian Operator
2.2.2 One-dimensional Position Operator and Eigenket
2.2.3 One-dimensional Momentum Operator and Eigenket
2.2.4 Commutator
2.2.5 Compatibility and Completeness
2.2.6 Unitary Operator2.3. Direct Product
Chapter 3. Harmonic Oscillator and Vibrational Spectroscopy
3.1 Schrödinger Equation
3.2 Operator Approach
3.3. General Time Dependent State
3.4 Vibrational Spectroscopy
Chapter 4. Multidimensional Systems and Separation of Variables
4.1 Position, Momentum, and Hamiltonian in Multidimensional Space
4.2 Particle in a Three-dimensional Rectangular Box
4.3 Center of Mass and Relative Coordinate System
Chapter 5. Rotational States and Spectroscopy
5.1 Rotation in Two-dimension
5.2 Rotation in Three-dimension
5.3 General Three-dimensional Rotation of a Diatomic Molecule
5.4 Rotational Spectroscopy
5.5 Ro-vibrational Transition
Chapter 6. Hydrogen-like Systems and Spin-Orbit States of Electron
6.1 Separation in Spherical Coordinate System
6.2 Energies and Spatial States of an Electron in Hydrogen-like Systems
6.3 Spin States of an Electron
Chapter 7. Approximation Methods
7.1 Variational Principle
7.1.1 General Proof
7.1.2 Simple Examples
7.1.3 Approximation with Linear Combination of Basis Sets
7.2 Time Independent Perturbation Theory
7.2.1 Non-degenerate Perturbation Theory and Examples
7.2.3 Degenerate Perturbation Theory and Examples
7.3 Time Dependent Perturbation Theory
7.3.1 General Derivation of Fermi’s Golden Rule
7.3.2 Super-Exchange Approximation
Chapter 8. Many-electron Systems and Atomic Spectroscopy
8.1 Spins and Pauli Principle
8.2 Aufbau Principle and Selection Rules for Atomic Spectroscopy
8.3 Helium Atom8.2.1 Self-consistent Field Approximation
8.2.2 Application of Perturbation Theory
8.4 Hartree-Fock Theory
8.5 Density Functional Theory
Chapter 9. Polyatomic Molecules and Molecular Spectroscopy
9.1 Born-Oppenheimer Approximation9.2 Example of H2
9.3 Conjugated Hydrocarbons and Hückel Approximation
9.4 Group Theory
9.5 Spectroscopy of Polyatomic Molecules
9.5.1 Infrared and Raman Spectroscopy
9.5.2 Linear Electronic Spectroscopy
Chapter 10. Electronic Structure Calculation Methods of Polyatomic Molecules
10.1 Hartree-Fock Theory
10.2 Configuration Interaction and Multi-reference methods
10.2 Density Functional Theory
10.3 Time Dependent Density Functional Theory
10.4 Green Function Approaches
Chapter 11. Path Integral Representation and Applications
11.1 Path Integral Representation of Real Time Propagator
11.2 Simple Examples
11.3 Path Integral Representation of Density Operator
Chapter 12. Quantum Dynamics Methods
12.1 Numerical Solution of Time Dependent Schrödinger Equation
12.2 Open System Quantum Dynamics Methods
12.2.1 Projection Operator Formalism
12.2.2 Stochastic Schrödinger Equation Approach
Chapter 13. Quantum Light
13.1 Second Quantization of Electromagnetic Field
13.2 Interaction of Molecules with Quantum Light
Chapter 14. Quantum Information, Control, Computation, and Sensing
Chapter 15. Conclusion and Open Issues for Research
Seogjoo J. Jang is a Professor of Chemistry at Queens College of the City University of New York (CUNY), and is a doctoral faculty member of both the Chemistry and Physics PhD programs at the Graduate Center of CUNY. He obtained his BS (1989) and MS (1993) degrees in Chemistry from Seoul National University, and a Ph.D. degree (1999) in Chemistry from the University of Pennsylvania. He then worked as a postdoctoral associate at MIT (1999-2002) and as a Goldhaber Distinguished Fellow (2003-2005) at Brookhaven National Laboratory before starting his faculty position at Queens College, CUNY in 2005. His research expertise is in quantum dynamics theories and computational modeling. In particular, he has pioneered modern theories of resonance energy transfer that are now being incorporated into theoretical analyses of experimental data on complex molecular systems and has made key contributions to understanding the role of delocalized excitons in photosynthetic light harvesting complexes. He is a recipient of the National Science Foundation CAREER Award (2009) and the Camille Dreyfus Teacher Scholar Award (2010).
Demystifies Dirac notation, helping students build a strong foundation in quantum mechanics
Introduces often-neglected topics such as path integrals, open system quantum dynamics, and Green’s functions
Provides an accessible guide to quantum theory without sacrificing rigor or completeness
Date de parution : 06-2023
Ouvrage de 432 p.
15.5x23.5 cm