Photoelectrochemical Solar Cells Advances in Solar Cell Materials and Storage (ASCMS) Series
Coordonnateurs : Sankir Nurdan Demirci, Sankir Mehmet
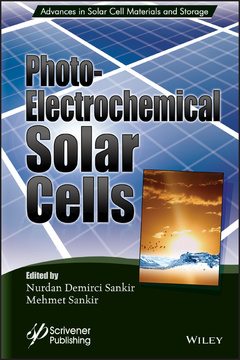
This book provides a broad overall view of the photoelectrochemical systems for solar hydrogen generation, and new and novel materials for photoelectrochemical solar cell applications.
Hydrogen has a huge potential as a safe and efficient energy carrier, which can be used directly in fuel cells to obtain electricity, or it can be used in the chemical industry, fossil fuel processing or ammonia production. However, hydrogen is not freely available in nature and it needs to be produced. Photoelectrochemical solar cells produce hydrogen from water using sunlight and specialized semiconductors, which use solar energy to directly dissociate water molecules into hydrogen and oxygen. Hence, these systems reduce fossil fuels dependency and curb carbon dioxide emissions.
Photoelectrochemical Solar Cells compiles the objectives related to the new semiconductor materials and manufacturing techniques for solar hydrogen generation. The chapters are written by distinguished authors who have extensive experience in their fields. Multidisciplinary contributors from physics, chemical engineering, materials science, and electrical and electronic information engineering, provide an in-depth coverage of the topic. Readers and users have the opportunity to learn not only about the fundamentals but also the various aspects of the materials science and manufacturing technologies for photoelectrochemical solar cells and the hydrogen generation systems via photoelectrochemical conversion.
This groundbreaking book features:
- Description of solar hydrogen generation via photoelectrochemical process
- Designs of photoelectrochemical systems
- Measurements and efficiency definition protocols for photoelectrochemical solar cells
- Metal oxides for solar water splitting
- Semiconductor photocatalysts
- Bismuth vanadate-based materials for solar water splitting
- Copper-based chalcopyrite and kesterite materials for solar water splitting
- Eutectic composites for solar water splitting
- Photocatalytic formation of composite electrodes
Preface xi
Part I: General Concepts and Photoelectrochemical Systems 1
1 Photoelectrochemical Reaction Engineering for Solar Fuels Production 3
Isaac Holmes-Gentle, Faye Alhersh, Franky Bedoya and Klaus Hellgardt
1.1 Introduction 3
1.1.1 Undeveloped Power of Renewables 4
1.1.2 Comparison Solar Hydrogen from Different Sources 5
1.1.3 Economic Targets for Hydrogen Production and PEC Systems 6
1.1.4 Goals of Using Hydrogen 8
1.2 Theory and Classification of PEC Systems 9
1.2.1 Classification Framework for PEC Cell Conceptual Design 10
1.2.2 Classification Framework for Design of PEC Devices 13
1.2.3 Integrated Device vs PV + Electrolysis 19
1.3 Scaling Up of PEC Reactors 19
1.4 Reactor Designs 20
1.5 Systems-Level Design 28
1.6 Outlook 30
1.6.1 Future Reactor Designs 30
1.6.1.1 Perforated Designs 30
1.6.1.2 Membrane-less and Microfluidic Designs 31
1.6.1.3 Redox-Mediated Systems 31
1.6.2 Avenues for Future Research 33
1.6.2.1 Intensification and Waste Heat Utilization 33
1.6.2.2 Usefulness of Oxidation and Coupled Process with Hydrogen Generation 33
1.7 Summary and Conclusions 34
References 35
2 The Measurements and Efficiency Definition Protocols in Photoelectrochemical Solar Hydrogen Generation 43
Jingwei Huang and Qizhao Wang
2.1 Introduction 43
2.2 PEC Measurement 44
2.2.1 Measurements of Optical Properties 44
2.2.2 Polarization Curve Measurements 45
2.2.3 Photocurrent Transients Measurements 46
2.2.4 IPCE and APCE Measurements 47
2.2.5 Mott–Schottky Measurements 48
2.2.6 Measurement (Calculation) of Charge Separation Efficiency 50
2.2.7 Measurements of Charge Injection Efficiency 51
2.8 Gas Evolution Measurements 52
2.3 The Efficiency Definition Protocols in PEC Water Splitting 53
2.3.1 Solar-to-Hydrogen Conversion Efficiency 53
2.3.2 Applied Bias Photon-to-Current Efficiency 54
2.3.3 IPCE and APCE 55
2.4 Summary 56
References 56
3 Photoelectrochemical Cell: A Versatile Device for Sustainable Hydrogen Production 59
Mohit Prasad, Vidhika Sharma, Avinash Rokade and Sandesh Jadkar
3.1 Introduction 60
3.2 Photoelctrochemical (PEC) Cells 61
3.2.1 Solar-to-Hydrogen (STH) Conversion Efficiency 65
3.2.2 Applied Bias Photon-to-Current Efficiency (ABPE) 65
3.2.3 External Quantum Efficiency (EQE) or Incident Photon-to-Current Efficiency (IPCE) 65
3.2.4 Internal Quantum Efficiency (IQE) or Absorbed Photon-to-Current Efficiency (APCE) 66
3.3 Monometal Oxide Systems for PEC H2 Generation 66
3.3.1 Titanium Dioxide (TiO2) 67
3.3.2 Zinc Oxide (ZnO) 68
3.3.3 Tungsten Oxide (WO3) 70
3.3.4 Iron Oxide (Fe2O3) 75
3.3.5 Bismuth Vandate (BiVO4) 76
3.4 Complex Nanostructures for PEC Splitting of Water 77
3.4.1 Plasmonic Metal Semiconductor Composite Photoelectrodes 77
3.4.2 Semiconductor Heterojunctions 80
3.4.3 Quantum Dots Sensitized Semiconductor Photoelectrodes 82
3.4.4 Synergistic Effect in Semiconductor Photoelectrodes 83
3.4.5 Biosensitized Semiconductor Photoelectrodes 85
3.4.6 Tandem Stand-alone PEC Water-Splitting Device 92
3.5 Conclusion and Outlook 98
Acknowledgments 101
References 101
4 Hydrogen Generation from Photoelectrochemical Water Splitting 121
Yanqi Xu, Qian Zhao, Cui Du, Chen Zhou, Huaiguo Xue and Shengyang Yang
4.1 Introduction 122
4.2 Principle of Photoelectrochemical (PEC) Hydrogen Generation 122
4.3 Photoeletrode Materials 125
4.3.1 Photoanode Materials 125
4.3.1.1 TiO2-Based Photoelectrode 125
4.3.1.2 BiVO4-Based Photoelectrode 126
4.3.1.3 α-Fe2O3-Based Photoelectrode 129
4.3.2 Photocathode Materials 129
4.3.2.1 Copper-Based Chalcogenides-Based Photoelectrode 129
4.3.2.2 Silicon-Based Photoelectrode 130
4.3.2.3 Cu2O-Based Photoelectrode 131
4.3.2.4 III-V Group Materials 132
4.3.2.5 CdS-Based Photoelectrode 134
4.4 Advances in Photoelectrochemical (PEC) Hydrogen Generation 135
4.4.1 Monocomponent Catalyst 135
4.4.2 Functional Cocatalyst 137
4.4.3 Z-scheme Catalyst 139
4.5 Pros and cons of photoelectrodes and photocatalysts 142
4.6 Conclusion and Outlook 144
Acknowledgments 145
References 145
Part II: Photoactive Materials for Solar Hydrogen Generation 159
5 Hematite Materials for Solar-Driven Photoelectrochemical Cells 161
Tianyu Liu, Martina Morelli and Yat Li
5.1 Introduction 161
5.2 Physical Properties of Hematite 163
5.2.1 Crystal Structure 163
5.2.2 Optical Properties 164
5.2.3 Electronic Properties 165
5.2.4 Band Structure 166
5.2.5 Overview of Hematite Bottlenecks and Corresponding Strategies 167
5.2.5.1 Addressing Poor Light Absorption Efficiency 168
5.2.5.2 Addressing Fast Charge Carrier Recombination 169
5.2.5.3 Addressing Sluggish Water Oxidation 5.3 Kinetics 169
5.3 Experimental Strategies to Enhance the Photoactivity of Hematite 170
5.3.1 Nanostructuring 170
5.3.1.1 Direct Synthesis 170
5.3.1.3 In Situ Structural Transformation 172
5.3.1.4 “Locking” Nanostructures 173
5.3.2 Doping 175
5.3.2.1 Oxygen Vacancies 175
5.3.2.2 Foreign Ion Doping 177
5.3.3 Construction of Heterojunctions 180
5.3.3.1 Semiconducting Overlayers 180
5.3.3.2 Sensitization and Tandem Cells 181
5.3.3.3 OER Catalysts 182
5.3.3.4 Engineering of Current Collectors 184
5.4 Fundamental Characteristics of the PEC Behaviors of Hematite 185
5.4.1 Transient Absorption Spectroscopy 185
5.4.2 Effects of Morphology 196
5.4.3 Effect of Doping 198
5.4.3.1 Oxygen (O) Vacancies 198
5.4.3.2 n-type Dopants 199
5.4.3.3 p-type Dopants 201
5.4.3.4 Isovalent Dopants 201
5.4.3.5 Multiple Dopants 201
5.4.4 Effect of Water Oxidation Catalysts 202
5.4.4.1 Mechanism of Uncatalyzed Water Oxidation 202
5.4.4.2 Mechanism of Catalyzed Water Oxidation 203
5.4.5 Effect of Heterojunctions 204
5.4.5.1 Facilitating Charge Separation and Transfer 204
5.4.5.2 Surface Passivation 206
5.4.5.3 Back-contact Engineering 207
5.5 Summary 208
References 209
6 Design of Bismuth Vanadate-Based Materials: New Advanced Photoanodes for Solar Hydrogen Generation 219
Olivier Monfort, Panagiotis Lianos and Gustav Plesch
6.1 Introduction 220
6.2 Photoanodes in Photoelectrochemical Processes 220
6.3 Bismuth Vanadate (BiVO4) 224
6.3.1 Structure and Properties of BiVO4 225
6.3.2 Synthesis of BiVO4 226
6.3.3 Applications of BiVO4 Materials 227
6.4 BiVO4 as Photoanode for Solar Hydrogen Generation 228
6.4.1 Optimization of the Photoanode 228
6.4.1.1 Photoanode Preparation 228
6.4.1.2 Choice of the Electrolyte 231
6.4.2 Solar Hydrogen Generation by Water Splitting 233
6.5 Modified BiVO4 Photoanodes 236
6.5.1 Transition Metal-Modified BiVO4 237
6.5.1.1 Generalities 237
6.5.1.2 Nb-modified BiVO4 238
6.5.2 BiVO4 Composites 240
6.5.2.1 Generalities 240
6.5.2.2 BiVO4/TiO2 Composite 242
6.6 Conclusion 245
6.7 Acknowledgments 246
References 246
7 Copper-Based Chalcopyrite and Kesterite Materials for Solar Hydrogen Generation 251
Cigdem Tuc Altaf, Nazrin Abdullayeva and Nurdan Demirci Sankir
7.1 Introduction 252
7.2 Chalcopyrite I-III-VI2 Semiconductors 253
7.2.1 Material Properties 253
7.2.2 Synthesis Techniques of Chalcopyrite CuInS/Se2 Nanocrystals 255
7.2.2.1 Hot-Injection Method 258
7.2.2.2 Heat-Up (Noninjection) Method 258
7.2.2.3 Thermal Decomposition Method 258
7.2.2.4 Solvothermal Method 259
7.2.2.5 Microwave Treatment Method 260
7.2.3 Chalcopyrite CuInS/Se2 Thin-Film Fabrication Methods 260
7.2.3.1 Vacuum-Based Techniques 262
7.2.3.2 Nonvacuum Techniques 263
7.2.4 Applications in Photoelectrochemical Cells 266
7.3 Cu-Based Kesterite (I2-II-IV-VI4) Semiconductors 269
7.3.1 Material Properties 269
7.3.2 Synthesis Techniques of Kesterite Cu2ZnSnS/Se4 Nanocrystals 272
7.3.2.1 Hot-Injection Method 272
7.3.2.2 Solvothermal/Hydrothermal Method 274
7.3.2.3 Microwave-Assisted Chemical Synthesis 275
7.3.2.4 Additional Novel Approaches to CZTS Nanocrystal Syntheses 275
7.3.3 Kesterite Cu2ZnSnS4 Thin-Film Fabrication Methods 277
7.3.3.1 Vacuum-based Techniques 277
7.3.3.2 Nonvacuum Techniques 280
7.3.4 Applications in Photoelectrochemical Cells 284
7.4 Concluding Remarks 284
References 287
8 Eutectic Composites for Photoelectrochemical Solar Cells (PSCs) 297
J. Sar, K. Kolodziejak, K. Wysmulek, K. Orlinski, A. Kusior, M. Radecka, A. Trenczek-Zajac, K. Zakrzewska and D.A. Pawlak
8.1 Introduction 297
8.2 The Photoelectrolysis of Water as a Source of Hydrogen 298
8.3 Experimental Methods for Studying Photoactive Materials Such as Electrochemical (Mott–Schottky Plots) and Photoelectrochemical Determination of the Flat-Band Potential, Impedance Spectroscopy, and Bandgap by Optical Spectroscopy 302
8.4 Eutectic Composites 318
8.5 Methods of Obtaining Eutectic Composites 322
8.6 Eutectic Composites used for Photoelectrochemical Water Splitting 324
8.7 Other Potential Eutectic Composites 328
8.8 Modification of the Properties of Eutectic Composites 329
8.9 Conclusions 331
References 332
Part III: Photoelectrochemical Related Systems 341
9 Implementation of Multijunction Solar Cells in Integrated Devices for the Generation of Solar Fuels 343
V. Smirnov, K. Welter, F. Finger, F. Urbain, J.R. Morante, B. Kaiser and W. Jaegermann
9.1 Introduction 344
9.2 Multijunction Solar Cells as Photoelectrodes 349
9.3 PV-EC Devices Based on Multijunction Solar Cells 355
9.4 Promising Device Designs, Future Prospects 362
9.5 Summary and Conclusions 367
References 370
10 Photoelectrochemical Cells: Dye-Sensitized Solar Cells 375
Go Kawamura, Pascal Nbelayim, Wai Kian Tan and Atsunori Matsuda
10.1 Introduction 376
10.2 Brief History of Solar Cells to DSSCs 377
10.3 Structure, Components, and Working Principle of the DSSC 377
10.3.1 The Transparent Conducting Oxide (TCO) Substrate 379
10.3.2 The Hole Blocking Layer (HBL) 379
10.3.3 The Photoanode 379
10.3.4 The Sensitizer/Dye 383
10.3.5 The HTM/Electrolyte 385
10.3.6 The CE 385
10.3.7 Electron Kinetics in an Active DSSC 386
10.4 Characterization Techniques for DSSCs 387
10.4.1 Computational Modeling 387
10.4.2 Morphological and Structural Studies 387
10.4.2.1 Electron Microscopy 387
10.4.2.2 X-Ray Diffraction 388
10.4.3 Dye Adsorption. 389
10.4.4 Spectroscopic Techniques 389
10.4.4.1 Optical (UV–Vis) Spectroscopy 389
10.4.4.2 X-ray Photoelectron Spectroscopy 390
10.4.4.3 FTIR Spectroscopy 390
10.4.4.4 Raman Spectroscopy 390
10.4.4.5 Material Composition 391
10.4.5 Electromagnetic Measurements 391
10.4.5.1 Hall Effect Measurement 391
10.4.5.2 Electron Paramagnetic Resonance Analysis 391
10.4.6 (Photo-)Electrochemical Measurements 391
10.4.6.1 Photovoltaic Properties 392
10.4.6.2 Electrochemical Impedance Spectroscopy 392
10.4.6.3 Electron Transport 392
10.4.6.4 Electron Lifetime 393
10.4.6.5 Electron Concentration 394
10.4.6.6 Flat-band Potential 394
10.4.6.7 Charge Collection Efficiency 394
10.5 Plasmonic DSSCs 395
10.6 Dye-Sensitized Solar Hydrogen Production 398
10.7 Applications and Future Outlook of DSSC 403
10.8 Academic 404
References 405
11 Photocatalytic Formation of Composite Electrodes for Semiconductor-Sensitized Solar Cells 415
Oleksandr Stroyuk, Andriy Kozytskiy and Stepan Kuchmiy
11.1 Introduction 416
11.2 Photocatalytic Deposition of Metal Sulfide Nanoparticles on the Surface of Wide-Bandgap Semiconductors 417
11.2.1 Photodeposition of Cadmium Sulfide NPs 420
11.2.2 Photocatalytic Deposition of Lead Sulfide 430
11.2.3 Photocatalytic Deposition of Silver Sulfide 431
11.2.4 Photodeposition of Antimony Sulfide 431
11.2.5 Photocatalytic Deposition of Molybdenum and Tungsten Sulfides 433
11.2.6 Photocatalytic Deposition of Copper Sulfide 434
11.3 Photocatalytic Deposition of Metal Selenides 435
11.4 Conclusion and Outlook 442
References 443
Index 449
Nurdan Demirci Sankir is currently an Associate Professor in the Materials Science and Nanotechnology Engineering Department at the TOBB University of Economics and Technology (TOBB ETU), Ankara, Turkey. She received her M.Eng and PhD degrees in Materials Science and Engineering from the Virginia Polytechnic and State University, USA in 2005. Nurdan has actively carried out research and consulting activities in the areas of photovoltaic devices, solution based thin film manufacturing, solar driven water splitting, photocatalytic degradation and nanostructured semiconductors.
Mehmet Sankir received his PhD in Macromolecular Science and Engineering from the Virginia Polytechnic and State University, USA in 2005. Dr. Sankir is currently an Associate Professor in the Department of Materials Science and Nanotechnology Engineering, TOBB University of Economics and Technology, Ankara, Turkey and group leader of Advanced Membrane Technologies Laboratory. Dr. Sankir has actively carried out research and consulting activities in the areas of membranes for fuel cells, flow batteries, hydrogen generation and desalination.
Date de parution : 01-2019
Ouvrage de 480 p.
1x1 cm
Thèmes de Photoelectrochemical Solar Cells :
Mots-clés :
hydrogen; photoelectrochemical; overall view; book; systems; broad; solar; cell; novel; applications; safe; carrier; energy; huge potential; efficient; electricity; directly; fuel; chemical industry; production; fossil; nature