Perovskites and Related Mixed Oxides Concepts and Applications
Auteurs : Granger Pascal, Parvulescu Vasile I., Kaliaguine Serge, Prellier Wilfrid
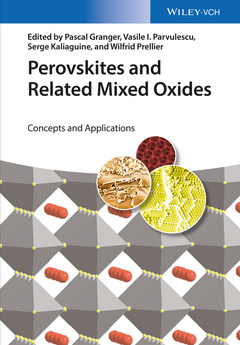
The first volume begins by summarizing the different synthesis routes from molten salts at high temperatures to colloidal crystal template methods, before going on to focus on the physical properties of the resulting materials and their related applications in the fields of electronics, energy harvesting, and storage as well as electromechanics and superconductivity. The second volume is dedicated to the catalytic applications of perovskites and related mixed oxides, including, but not limited to total oxidation of hydrocarbons, dry reforming of methane and denitrogenation. The concluding section deals with the development of chemical reactors and novel perovskite–based applications, such as fuel cells and high–performance ceramic membranes. Throughout, the contributions clearly point out the intimate links between structure, properties and applications of these materials, making this an invaluable tool for materials scientists and for catalytic and physical chemists.
List of Contributors XXIII
Preface XXXV
Volume 1 Part One Rational Design and Related Physical Properties 1
1 From Solid–State Chemistry to Soft Chemistry Routes 3
Vicente Rives
1.1 Introduction 3
1.2 Processes Involving Solids 4
1.2.1 The Ceramic Method 4
1.2.2 Microwave Synthesis 5
1.2.3 Self–Propagating High–Temperature Synthesis (SHS) 6
1.2.4 The Precursor Method 6
1.2.5 Hydrothermal Synthesis 7
1.2.6 High–Pressure Methods 8
1.2.7 Mechanochemistry 8
1.2.8 Other Methods Starting from Solids 9
1.3 Processes Involving Liquids 9
1.3.1 Flux Method 9
1.3.2 Molten Salt Electrolysis 10
1.3.3 Sol Gel 10
1.3.4 Spray Drying (SD) and Related Methods 13
1.3.4.1 Freeze–Drying 14
1.3.4.2 Spray Freeze–Drying 14
1.3.5 Molecular Self–Assembling 14
1.3.6 Other Methods Starting from Liquid Reactants or Solutions 15
1.3.6.1 Ionic Liquids 15
1.3.6.2 The Gel Combustion Method 15
1.3.6.3 Sonication 15
1.3.6.4 Reverse Microemulsion 15
1.4 Processes Involving Gases or Vapors 16
1.4.1 Gas Flame Combustion 16
1.4.2 Chemical Vapor Deposition (CVD) 16
1.5 Single Crystals 16
1.6 Nanoparticles 18
1.7 Films 19
1.8 Conclusions 19
References 20
2 Mechanochemistry 25
Houshang Alamdari and Sébastien Royer
2.1 Introduction 25
2.2 Historical Development 25
2.3 Terminology 28
2.4 Mechanosynthesis Process 29
2.5 Milling Facilities 32
2.5.1 Spex Mills 32
2.5.2 Planetary Mills 34
2.5.3 Attrition Mills 35
2.5.4 Zoz Mills 36
2.6 Mechanosynthesis of Perovskites 37
2.6.1 Looking for an Alternative Route to Synthesize New Compositions 38
2.6.2 Lowering Sintering Temperature 38
2.6.3 Reducing Crystallite Size and Modifying Particle Morphology 39
2.6.4 Increasing Specific Surface Area 40
2.7 Concluding Remarks 42
References 43
3 Synthesis and Catalytic Applications of Nanocast Oxide–Type Perovskites 47
Mahesh Muraleedharan Nair and Serge Kaliaguine
3.1 Introduction 47
3.2 Perovskite Structure 48
3.3 Evolution of Perovskite Synthesis 49
3.4 General Principles of Nanocasting 51
3.5 Nanocasting of Perovskites 52
3.6 Catalytic Studies 56
3.6.1 Total Oxidation of Methane 56
3.6.2 Reduction of NO to N2 57
3.6.3 Chemical Looping Combustion 58
3.6.4 Total Oxidation of Methanol 59
3.6.5 Dry Reforming of Methane 60
3.7 Conclusions and Perspectives 63
References 64
4 Aerosol Spray Synthesis of Powder Perovskite–Type Oxides 69
Davide Ferri, Andre Heel, and Dariusz Burnat
4.1 Introduction 69
4.2 Flame Spray Synthesis 71
4.2.1 Methane Flame 72
4.2.2 Acetylene Flame 75
4.3 Flame Hydrolysis 80
4.4 Ultrasonic Spray Synthesis 82
4.4.1 General Particle Properties 83
4.4.2 Citric Acid Assisted Synthesis 85
References 87
5 Application of Microwave and Ultrasound Irradiation in the Synthesis of Perovskite–Type Oxides ABO3 91
Juan C. Colmenares, Agnieszka Magdziarz, and Pawe³ Lisowski
5.1 Introduction 91
5.2 Microwave Methodology 92
5.2.1 Basic Concepts of Microwave Chemistry 92
5.2.2 Microwave Heating in Combination with Traditional Synthesis Methods 93
5.2.2.1 Microwave–Assisted Hydrothermal Method (HTMW) 93
5.2.2.2 Other Microwave–Assisted Methods 100
5.3 Ultrasound Methodology 101
5.3.1 Basic Concepts of Ultrasound Chemistry 101
5.3.2 Ultrasound–Assisted Coprecipitation Method 102
5.3.3 Ultrasound–Assisted Sol Gel Method 103
5.3.4 Ultrasound Spray Pyrolysis 105
5.3.5 Other Ultrasound–Assisted Methods 107
5.4 Concluding Remarks and Outlook 108
Acknowledgments 108
References 109
6 Three–Dimensionally Ordered Macroporous (3DOM) Perovskite Mixed Metal Oxides 113
Masahiro Sadakane and Wataru Ueda
6.1 Introduction 113
6.2 3DOM Materials 114
6.2.1 Preparation of 3DOM Materials 114
6.2.1.1 Colloidal Crystal Templates 114
6.2.1.2 Infiltration of Precursors in the Voids of Templates 122
6.2.1.3 Removal of Templates 122
6.2.2 Structure of 3DOM Materials (Inverse Opal Structures) 122
6.3 Preparation of 3DOM Perovskite Mixed Metal Oxides 123
6.3.1 Precursor Solution 123
6.3.2 Selection of Sphere Templates 126
6.3.3 Synthesis Methods and Applications of 3DOM Perovskite Mixed Metal Oxides 127
6.3.4 Preparation of 3DOM LaFeO3 with Different Pore Sizes 131
6.3.4.1 Preparation of Polymer Spheres and Colloidal Crystal Templates 131
6.3.4.2 Synthesis of 3DOM LaFeO3 134
6.3.4.3 Characterization of 3DOM LaFeO3 134
6.3.4.4 Formation Mechanism 136
6.4 Conclusions 138
References 138
7 Thin Films and Superlattice Synthesis 143
Carmela Aruta and Antonello Tebano
7.1 Introduction 143
7.2 Thin Films and Superlattices Growth 145
7.2.1 Deposition Techniques 145
7.2.1.1 MBE 145
7.2.1.2 PLD 149
7.2.1.3 Sputtering 153
7.2.2 In Situ Monitoring: RHEED and Plume Analysis 156
7.2.2.1 RHEED 156
7.2.2.2 Plume Analysis 159
7.3 Concluding Remarks 162
Acknowledgments 162
References 162
8 Perovskite and Derivative Compounds as Mixed Ionic Electronic Conductors 169
Caroline Pirovano, Aurélie Rolle, and Rose–Noëlle Vannier
8.1 Introduction 169
8.2 Perovskite as Mixed Ionic Electronic Conductors 170
8.2.1 The Perovskite: A Flexible Structure for Mixed Ionic Electronic Conductivity 170
8.2.2 Cobaltites: Among the Best MIEC Materials 173
8.2.3 MIEC Electrochemical Performances as SOFC or SOEC Electrodes 173
8.3 Conductivity and Oxygen Transport Properties in Mixed Ionic– and Electronic–Conducting Perovskites 176
8.3.1 Electrical Conductivity 177
8.3.2 Diffusion Coefficients 177
8.3.3 Surface Exchange Coefficients 179
8.3.4 Perovskite Materials and Related Compounds Oxygen Transport Parameters 180
8.4 Conclusions 183
References 184
9 Perovskite and Related Oxides for Energy Harvesting by Thermoelectricity 189
Sascha Populoh, O. Brunko, L. Karvonen, L. Sagarna, G. Saucke, P. Thiel, M. Trottmann, N. Vogel–Schäuble, and A. Weidenkaff
9.1 Introduction to Thermoelectricity 189
9.2 CaMnO3–Based Compounds 190
9.3 EuTiO3 and Related Compounds 196
9.4 SrCoO3 and Related Phases 199
9.5 ZnO for Thermoelectric Applications 200
9.6 Thermoelectric Oxide Modules and Their Characterization 202
9.7 Concluding Remarks 204
References 204
10 Piezoelectrics and Multifunctional Composites 211
Ranjith Ramadurai and Vijayanandhini Kannan
10.1 History 211
10.2 Piezoelectricity: An Introduction 211
10.3 Piezoelectric Materials: An Overview 214
10.4 Lead–Free Piezoelectrics 215
10.4.1 BaTiO3 CaTiO3 BaZrO3 Solid Solutions 216
10.4.2 Structural Phase Diagram of BZT BCT 217
10.4.3 Piezoelectric Properties of BCT BZT 218
10.4.4 (Na0.5Bi0.5)TiO3 219
10.5 Piezoelectric Polymers 221
10.5.1 Polyvinylidene Fluoride 222
10.6 Piezoelectric Composites 223
10.7 Polymer Ceramic Hybrid Piezoelectric Composites 225
10.8 Multifunctional Piezoelectric Composites 226
10.9 Summary 229
References 230
11 Microstructure and Nanoscale Piezoelectric/Ferroelectric Properties in Ln2Ti2O7 (Ln = Lanthanide) Thin Films with Layered Perovskite Structure 233
Sébastien Saitzek, ZhenMian Shao, Alexandre Bayart, Pascal Roussel, and Rachel Desfeux
11.1 Introduction and Overview of Layered Perovskite Structures 233
11.2 Ln2Ti2O7 Compounds 236
11.2.1 Structural Properties of Ln2Ti2O7 with Ln = Lanthanide 236
11.2.2 Synthesis Way 237
11.2.3 Scope and Properties of the Ln2Ti2O7 Oxides 238
11.3 Growth and Structural Characterization of Ln2Ti2O7 Thin Films 239
11.3.1 Growth on (100)–Oriented SrTiO3 Substrates 239
11.3.2 Growth on (110)–Oriented SrTiO3 Substrates 242
11.3.3 Limit of Stability of the Layered Perovskite Structure 243
11.4 Piezo– and Ferroelectric Properties of Ln2Ti2O7 Thin Films 244
11.4.1 Experimental Setup 244
11.4.2 Ln2Ti2O7 (Ln = La, Pr, and Nd) Thin Films Grown on (110)–Oriented SrTiO3 Substrates 246
11.4.3 Ln2Ti2O7 (Ln = La, Pr, and Nd) Thin Films Grown on (100)–Oriented SrTiO3 Substrates 247
11.4.4 Metastable Ln2Ti2O7 (Ln = Sm, Eu, and Gd) Thin Films Grown on (110)–Oriented SrTiO3 Substrates 249
11.5 Conclusion 250
Acknowledgments 251
References 251
12 Pigments Based on Perovskite 259
Matteo Ardit, Giuseppe Cruciani, Michele Dondi, and Chiara Zanelli
12.1 Introduction 259
12.2 Perovskite Pigments 259
12.2.1 Red and Orange 261
12.2.2 Yellow 261
12.2.3 Brown to Light Brown 262
12.2.4 Magenta to Pink 263
12.2.5 Blue 263
12.2.6 Black 263
12.3 (Y, REE) Aluminate Perovskites: Crystal Chemistry and Structural Principles 263
12.3.1 Crystal Structure of Ideal and Distorted Ternary ABO3 Perovskites 263
12.3.2 Lattice Parameters, A Site Coordination, and Bond Valence Analysis in (Y,REE) Orthoaluminates 264
12.3.3 Tilting of Octahedral Framework and Tolerance Factor 268
12.4 Chromium Incorporation: Basic Concepts and the YAlO3 YCrO3 Case Study 269
12.4.1 Local Bond Distances 269
12.4.2 Structural Relaxation Coefficient 270
12.4.3 Comparison with Other Al Cr Solid Solutions 271
12.4.4 Polyhedral Bond Valence Method 272
Case Study 274
12.5 Origin of Color in (Y, REE) Orthoaluminates 279
References 284
13 Electrolyte Materials 289
Viorica Parvulescu
13.1 Introduction 289
13.2 Properties of Solid Electrolyte Materials 290
13.2.1 Synthesis Methods and Properties of Mixed Oxides Electrolytes 290
13.2.2 The Crystalline Phases and Conductivity 294
13.3 Mixed Oxides with Ionic Conductivity 295
13.3.1 Solid Electrolytes Based on ZrO2 296
13.3.2 Solid Electrolytes Based on CeO2 298
13.4 Mixed Oxides with Mixed Conductivity 301
13.5 Applications of Mixed Oxides as Electrolytes and Mixed Conductors 303
13.6 Conclusions 306
References 306
14 CO2 Capture Using Dense Perovskite Membranes: Permeation Models 311
Marc Pera–Titus
14.1 MIEC Membranes for Gas Separation 311
14.2 Background for Mass Transfer Modeling in Perovskite Membranes 312
14.3 Gas Permeation Models for Perovskite Membranes 315
14.3.1 Single–Phase Perovskite Membranes 316
14.3.1.1 Models for O2 Semipermeation 318
14.3.1.2 Models for H2 Semipermeation 322
14.3.2 Dual–Phase Perovskite Membranes 325
14.3.2.1 Models for H2 Semipermeation within Supported Ni/Perovskite DFMs 326
14.3.2.2 Models for H2 Semipermeation in Ni–Cermets DFMs 326
14.3.2.3 Models for CO2 Semipermeation in Infiltrated MC/Perovskite DPMs 327
14.4 Measurement of Diffusion and Surface Exchange Coefficients 329
14.4.1 Semipermeation Coupled to Electrical Potential Measurements 329
14.4.2 Isotopic Exchange Depth Profile (IEDP) 331
14.4.3 Electrical Conductivity Relaxation (ECR) 333
14.4.4 Electrochemical Impedance Spectroscopy (EIS) 333
14.4.5 Diffusion and Surface Exchange Coefficients: Structure Property Correlations 334
14.5 Conclusions 334
Glossary 335
Greek Symbols 336
Subscripts 336
Superscripts 337
Acronyms 337
References 337
15 Introduction to Rational Molecular Modeling Approaches 343
Randy Jalem and Masanobu Nakayama
15.1 Introduction 343
15.2 Theoretical Background on Ab Initio Calculation 343
15.2.1 Brief Review of Elementary Quantum Chemistry 343
15.2.2 Density Functional Theory 346
15.3 Simulation Model Construction 347
15.4 Electronic Structure 349
15.5 Ionic Transport 351
15.6 Atomic Arrangement, Phase Stability, and Transition 354
15.7 Conclusions and Outlook 359
References 360
Volume 2
Part Two Perovskite and Related Mixed Oxides in Catalysis: From the Structure to the Catalytic Properties 367
16 Methane Combustion on Perovskites 369
Athanasios Ladavos and Philippos Pomonis
16.1 Perovskites as a Diverse and Active Class of Materials 369
16.1.1 Structural Diversity, Tolerance Factor, and Thermodynamic Stability 370
16.2 Mixed Valences in Perovskites 371
16.2.1 Mixed Valences Due to Anion Deficiencies 371
16.2.2 Mixed Valences Due to Isostructural Substitution of Cations 373
16.3 The Reversed Uptake of Oxygen and Its Different Sources 373
16.4 The Mechanism of Methane Combustion 376
16.5 Kinetics of Methane Combustion 378
16.5.1 Rideal Eley kinetics 379
16.5.2 First–Order Kinetics 380
16.5.3 The Power Law Kinetics 384
16.5.4 The Two Term Kinetics 385
16.6 Conclusions 386
Acknowledgments 387
References 387
17 Total Oxidation of Volatile Organic Compounds 389
Vasile I. Parvulescu
17.1 Introduction 389
17.2 Specificity of Perovskites for Total Oxidation of VOCs 391
17.3 Morphology of Perovskites Investigated for Total Oxidation of VOCs 395
17.4 Total Oxidation of VOCs under Thermal Activation Conditions 397
17.5 Total Oxidation of Light Hydrocarbons 399
17.6 Total Oxidation of Oxygenated Organic Compounds 401
17.7 Total Oxidation of Halogenated Organic Compounds 402
17.8 Total Oxidation under Plasma Activation Conditions in Gas 404
17.9 Photocatalytic Destruction of VOC 406
17.10 Conclusions 407
References 408
18 Total Oxidation of Heavy Hydrocarbons and Aromatics 413
Vasile I. Parvulescu and Pascal Granger
18.1 Introduction 413
18.2 Perovskites and Oxygen Vacancy 414
18.3 Total Oxidation under Thermal Activation Conditions 416
18.4 Total Oxidation of Aromatic Hydrocarbons 417
18.5 Total Oxidation of Polycyclic Aromatic Hydrocarbons 424
18.6 Total Oxidation of Soot 425
18.7 Total Oxidation of Halogenated Hydrocarbons 426
18.8 Total Oxidation under Plasma Activation Conditions 428
18.9 Total Oxidation of Aromatics 429
18.10 Total Oxidation of Soot 431
18.11 Conclusions 431
References 432
19 Progresses on Soot Combustion Perovskite Catalysts 437
Agustín Bueno–López
19.1 Introduction 437
19.2 Particular Aspects of the Soot Combustion Reactions 438
19.3 Soot Combustion Perovskite Catalysts: Effect of Partial Substitution of Cations in the Perovskite Oxide 439
19.4 Kinetic and Mechanistic Studies 442
19.5 Three–Dimensionally Ordered Macroporous Soot Combustion Perovskite Catalysts 444
19.6 Study of Soot Combustion Perovskite Catalysts in Real Diesel Exhausts 445
19.7 Microwave–Assisted Perovskite–Catalyzed Soot Combustion 446
19.8 Deactivation of Soot Combustion Catalysts by Perovskite Structure Formation 446
19.9 Conclusions 446
Acknowledgments 447
References 447
20 Low–Temperature CO Oxidation 451
Oscar H. Laguna, Luis F. Bobadilla, Willinton Y. Hernández, and Miguel Angel Centeno
20.1 Overview 451
20.2 Low–Temperature CO Oxidation Reaction 453
20.2.1 LaBO3–Type Perovskites 454
20.2.3 Noble Metal Perovskite Hybrid Materials 456
20.3 H2 Purification–Related CO Oxidations: Water–Gas Shift (WGS) and PROX Reactions 459
20.3.1 Perovskites for the Water–Gas Shift Reaction 460
20.3.2 Perovskites for the Preferential CO Oxidation in the Presence of H2 (PROX) 464
20.4 Concluding Remarks 468
Acknowledgments 468
References 468
21 Liquid–Phase Catalytic Oxidations with Perovskites and Related Mixed Oxides 475
Viorica Parvulescu
21.1 Introduction 475
21.2 Active Sites and Oxidants 476
21.3 Catalytic Reactions with Green Oxidants 480
21.3.1 Perovskites Catalysts 480
21.3.2 Microporous Mixed Oxide Catalysts 483
21.3.3 Mesoporous Mixed Oxide Catalysts 486
21.4 Heterogeneous Photo–Fenton Oxidation 488
21.4.1 Photo–Fenton Reactions with Perovskites 490
21.4.2 Photo–Fenton Reactions with Porous Mixed Oxides 491
21.5 Photocatalytic Ozonation Reactions 492
21.6 Conclusions 493
References 494
22 Dry Reforming of Methane 501
Catherine Batiot–Dupeyrat
22.1 Introduction 501
22.2 LaNiO3 as Catalyst Precursor for Carbon Dioxide Reforming of Methane 502
22.5 Perovskite as Support of Active Sites in the Dry Reforming of Methane 510
22.6 Supported Perovskite for Dry Reforming of Methane 510
22.7 Conclusion 512
References 512
23 Recent Progress in Oxidative Conversion of Methane to Value–Added Products 517
Evgenii V. Kondratenko and Uwe Rodemerck
23.1 Methane: Sources and Feedstock for Chemical Industry 517
23.2 Oxidative Coupling of Methane 519
23.2.1 OCM Reactors and Modes of Operation 520
23.2.2 OCM Process Concepts 522
23.2.3 Strategies for Developing New OCM Catalysts 526
23.3 Methane to Methanol and Its Derivatives 528
23.4 Methane to Acetic Acid 530
23.5 Conclusions 532
References 533
24 Steam Reforming of Alcohols from Biomass Conversion for H2 Production 539
Florence Epron, Nicolas Bion, Daniel Duprez, and Catherine Batiot–Dupeyrat
24.1 Introduction 539
24.2 Generalities on Alcohol Steam Reforming 539
24.2.1 Types of Alcohols Used 539
24.2.2 Reactions Involved and Thermodynamic Data 540
24.2.2.1 Ethanol Steam Reforming 540
24.2.2.2 Glycerol Steam Reforming 542
24.3 Catalysts 544
24.3.1 Types of Catalysts Used 544
24.3.1.1 Noble Metal Catalysts 545
24.3.1.2 Non–Noble Metal Catalysts 545
24.3.1.3 Effect of the Support 546
24.3.2 Why Perovskite–Type Catalysts are Good Candidates? 547
24.3.3 General Assessement 549
24.4 Catalytic Performances of Perovskite–Type Catalysts for H2 Production from Alcohols 549
24.4.1 Ethanol Steam Reforming 549
24.4.2 Glycerol Steam Reforming 551
24.5 Summary and Outlook 552
References 553
25 Three–Way Catalysis 559
Ioannis V. Yentekakis and Michalis Konsolakis
25.1 Three–Way Catalytic Converters (TWCs): An Introduction 559
25.2 Three–Way Catalytic Materials: Potentials/Aptitudes, Limitations, and Future Trends 563
25.3 Three–Way Catalysis by Ceria and Ceria–Based Mixed Oxides 565
25.3.1 CO Oxidation 567
25.3.2 Oxidation of Hydrocarbons 568
25.3.3 NO Reduction by CO or HCs 568
25.3.4 Simulated Stoichiometric Exhaust Conditions 568
25.4 Application of Perovskites in Exhaust Emission Control 570
25.4.1 Model Reactions 572
25.4.1.1 CO Oxidation 572
25.4.1.2 N2O Decomposition 573
25.4.1.3 NO Reduction by CO 573
25.4.1.4 NO Reduction by Propene 575
25.4.2 Simulated Exhaust Conditions 576
25.5 Conclusions and Guidelines 579
References 580
26 Lean Burn DeNOx Applications: Stationary and Mobile Sources 587
Angelos M. Efstathiou and Vasilis N. Stathopoulos
26.1 Scope 587
26.2 Introduction 588
26.2.1 Hydrogen–Selective Catalytic Reduction (H2–SCR) 588
26.2.2 Lean NOx After Treatment of Diesel Engine Emissions 590
26.3 Case Studies 594
26.3.1 H2–SCR of NO 594
26.3.2 Lean NOx Trap 601
26.3.3 Simultaneous NOx Reduction and Soot Oxidation 605
26.4 Concluding Remarks 605
References 606
27 Catalytic Abatement of N2O from Stationary Sources 611
Pascal Jean–Philippe Dacquin and Christophe Dujardin
27.1 Introduction 611
27.2 The Abatement of N2O From Nitric Acid Plant: A Case Study 613
27.2.1 Different Possible Scenarios 613
27.2.2 High–Temperature Decomposition of N2O 615
27.2.3 Medium–Temperature Decomposition of N2O 618
27.2.4 End–of–Pipe Technologies 622
27.3 Conclusion 626
References 627
28 Perovskites as Catalyst Precursors for Fischer Tropsch Synthesis 631
Anne–Cécile Roger and Alain Kiennemann
28.1 Introduction 631
28.2 Alcohols Synthesis 632
28.2.1 Methanol Synthesis 633
28.2.2 Higher Alcohols Synthesis 638
28.2.2.1 Ethanol Synthesis 638
28.2.2.2 C1 Cn Alcohols Synthesis 639
28.3 Hydrocarbons Synthesis 644
28.4 Conclusions 654
References 654
29 FexZr1 xO2 and Ce1 xFexO2 Mixed Oxide Catalysts: DRIFTS Analyses of Synthesis Gas and TPSR of Propane Dry Reforming 659
Rodrigo Brackmann, Ricardo Scheunemann, Andre Luiz Alberton, and Martin Schmal
29.1 Introduction 659
29.2.1.1 CO Adsorption 661
29.2.1.2 Adsorption of CO+O2+He 663
29.2.1.3 Adsorption of CO+O2+H2+He 664
29.2.2.1 Thermodynamics 667
29.2.2.2 Temperature–Programmed Surface Reaction 667
29.3 Conclusions 671
References 672
30 Photocatalytic Assisted Processes 675
Bogdan Cojocaru and Vasile I. Parvulescu
30.1 Introduction 675
30.2 Titanates 677
30.2.1 Calcium Titanates 677
30.2.2 Strontium Titanates 678
30.2.3 Barium Titanates 683
30.2.4 Lanthanum Titanates 684
30.2.5 Iron Titanates 685
30.2.6 Other Titanates 685
30.2.7 Bismuth Titanates 686
30.3 Ferrites 686
30.3.1 Calcium Ferrites 686
30.3.2 Strontium Ferrites 686
30.3.3 Barium Ferrites 687
30.3.4 Yttrium Ferrites 687
30.3.5 Rare Earth Ferrites 688
30.3.6 Other Ferrites 689
30.4 Conclusions 690
References 690
Part Three Future Prospects from Synthesis to Reactor Design 699
31 Mesoporous TM Oxide Materials by Surfactant–Assisted Soft Templating 701
Altug S. Poyraz, Yongtao Meng, Sourav Biswas, and Steven L. Suib
31.1 Introduction 701
31.1.1 Use of a Hard Template 701
31.1.2 Mesoporous Oxide Materials by Chemical Transformation 702
31.1.3 Mesoporous Oxide Materials by Soft Micelle Templating 703
31.2 Surfactant and Micelleization 705
31.2.1 Types of Surfactants 705
31.2.2 Inorganic Additives 705
31.2.3 Organic Additives 706
31.3 Surfactant Inorganic (S I) Interactions 707
31.3.1 Thermodynamics of Mesostructured Materials 707
31.3.2 Surfactant Inorganic ( Ginter) Interactions 707
31.3.2.1 Coulombic S I Interactions for Mesoporous TM Oxides 708
31.3.2.2 Covalent S I Interactions for Mesoporous TM Oxides 709
31.3.2.3 S to I Charge Transfer Interactions for Mesoporous TM Oxides 710
31.3.2.4 Hydrogen–Bonding (S I) Interactions for Mesoporous TM Oxides 711
31.4 Stability of a Mesoporous TM Oxide 712
31.4.1 Template Removal 713
31.5 Summary and Future Prospects 713
References 714
32 Development of Robust Mixed–Conducting Membranes with High Permeability and Stability 719
Tomás Ramirez–Reina, José Luis Santos, Nuria García–Moncada, Svetlana Ivanova, and José Antonio Odriozola
32.1 Overview 719
32.2 Mechanical Robustness 721
32.3 Chemical Robustness 725
32.3.1 Tolerance Toward CO2 725
32.3.2 Tolerance Toward SO2 729
32.3.3 Tolerance Toward Reducing Environments 731
32.4 Future Applications 732
References 732
33 Catalytic Reactors with Membrane Separation 739
Fausto Gallucci and Jon Zuniga
33.1 Introduction 739
33.2 Types of Reactors 740
33.2.1 Packed Bed Membrane Reactors 740
33.2.2 Fluidized Bed Membrane Reactors 744
33.3 Membranes for O2 Separation 753
33.3.1 Membrane Sealing 755
33.4 Membrane Reactors with O2 Membranes 758
33.5 Conclusions 768
References 768
34 The Development of Millistructured Reactors for High Temperature and Short Time Contact 773
Ana Raquel de la Osa, Anne Giroir–Fendler, and Jose Luis Valverde
34.1 Introduction 773
34.2 Classification of Microreactors 774
34.2.1 Capacity 775
34.2.2 Material 775
34.2.3 Reaction Phase 776
34.2.3.1 Reactions Involving Liquids 776
34.2.3.2 Gas Phase 776
34.2.3.3 Catalytic Reactions Involving Three Phases 777
34.2.4 Catalytic System 777
34.2.5 Other Configurations 778
34.3 Applications and Possible Scale–up 778
34.3.1 Ammonia Oxidation 779
34.3.2 Diesel Particulate Combustion 779
34.3.3 Ethylene Oxide Synthesis 779
34.3.4 Oxidative Coupling of Methane 779
34.3.5 Hydrogenation Reactions 780
34.3.5.1 Hydrogenation of Benzene to Cyclohexene 780
34.3.5.2 Hydrogenation of Cyclohexene 780
34.3.6 Dehydrogenation Reactions 780
34.3.6.1 Dehydrogenation of Methylcyclohexane 780
34.3.6.2 Dehydrogenation of Cyclohexane 780
34.3.6.3 Oxidative Dehydrogenation of Methanol 781
34.3.6.4 Dehydrogenation of Alkanes 781
34.3.7 Synthesis Gas Production 781
34.3.7.1 Steam Methane Reforming 781
34.3.7.2 Partial Oxidation of Methane 781
34.3.8 Fuel Production 781
34.3.8.1 Direct Partial Oxidation of Methane to C1 Oxygenates 781
34.3.8.2 Total Syngas Methanation to Synthetic Natural Gas 782
34.3.8.3 Fischer Tropsch Synthesis 782
34.3.8.4 Synthesis of Methanol and Ethanol 783
34.3.8.5 Synthesis of Dimethyl Ether 783
34.3.8.6 Biodiesel Production 783
34.3.8.7 Hydrogen Production 784
34.4 Simulation Case 785
34.5 Conclusions 789
References 791
35 Single Brick Solution for Lean–Burn DeNOx and Soot Abatement 797
Sonia Gil, Jesus Manuel Garcia–Vargas, Leonarda F. Liotta, Philippe Vernoux, and Anne Giroir–Fendler
35.1 Introduction 797
35.2 Diesel Posttreatment 799
35.2.1 Specificity of Diesel Engine 799
35.2.2 Diesel Unburned Hydrocarbon and Carbon Monoxide Oxidation 799
35.2.3 Treatment of Soot 801
35.2.4 DeNOx Reduction 803
35.2.4.1 Urea and NH3 Selective Catalytic Reduction 804
35.2.4.2 Single Brick Solution for Lean–Burn DeNOx and Soot Abatement 807
35.3 Conclusion 810
References 811
36 Tools for the Kinetics of Fast Reactions 817
Gregory Biausque, Marie Rochoux, David Farrusseng, and Yves Schuurman
36.1 Introduction 817
36.2 Oxygen Interaction 817
36.2.1 Oxygen Nonstoichiometry 818
36.2.2 Oxygen Isotopic Exchange Techniques 819
36.2.3 Secondary Ion Mass Spectrometry 819
36.2.4 Steady–State Isotopic Transient Oxygen Exchange 819
36.2.5 Case Study: Prediction of the Oxygen Permeation Flux through a Thin Ceramic Membrane from Powder Measurements 820
36.2.6 Conclusions 823
36.3 Measurement of Kinetics of Fast Reactions 823
36.3.1 Annular Reactor 824
36.3.2 Modeling of Annular Reactors 825
36.3.3 Case Study: Kinetics of High–Temperature Ammonia Oxidation in an Annular Reactor 827
36.3.4 TAP Reactor 830
36.3.5 Case Study: TAP Experiments for Ammonia Oxidation over LaCoO3 831
36.3.6 Conclusions 833
References 833
37 Perovskites as Oxygen Carrier–Transport Materials for Hydrogen and Carbon Monoxide Production by Chemical Looping Processes 839
Lori Nalbandian and Vassilis Zaspalis
37.1 Introduction 839
37.1.1 Chemical Looping Combustion 839
37.1.2 Oxygen Carriers 840
37.1.3 Chemical Looping Reforming 841
37.1.4 Chemical Looping Water Splitting and Chemical Looping Carbon Dioxide Splitting 842
37.1.5 Thermochemical Water or Carbon Dioxide Splitting 842
37.1.6 Chemical Looping in Dense Membrane Reactors 843
37.2 Perovskites for H2 and CO Production by Chemical Looping Processes 844
37.2.1 Powdered Perovskites: Chemical Looping Processes 845
37.2.1.1 Reduction by an Oxidizable Compound 845
37.2.1.2 Reduction by Solar Radiation 849
37.2.2 Perovskites as Dense Membranes 850
37.2.3 Perovskites Used as Supports 856
37.3 Conclusions 857
References 857
38 Perovskites and Related Mixed Oxides for SOFC Applications 863
Steven S.C. Chuang and Long Zhang
38.1 Introduction 863
38.2 Fuel Cells 864
38.3 Perovskites 870
38.3.1 Perovskite as a Cathode Material 870
38.3.2 Low–Temperature Cathodes 873
38.4 Anode Materials 874
38.5 Summary and Future R&D 875
References 876
39 Perovskite Membranes for CO2 Capture: Current Trends and Future Prospects 881
Marc Pera–Titus and Anne Giroir–Fendler
39.1 Introduction 881
39.2 Pre–, Post–, and Oxy–combustion CO2 Capture: High– versus Low–Temperature Membrane Technologies 882
39.2.1 Low–Temperature Membranes: Porous Inorganic Membranes 883
39.2.2 High–Temperature Membranes: Mixed Ionic Electronic Conducting Membranes Based on Perovskites 885
39.3 R&D Membrane Concepts for High–Temperature CO2 Capture 889
39.3.1 Perovskite Membranes for O2 Separation 889
39.3.1.1 O2 Separation and Combustion 889
39.3.1.2 Gasification Systems Combined with Combustion 890
39.3.2 Perovskite Membranes for H2 Separation and Steam Dosing 891
39.3.3 Perovskite–Containing Membranes for CO2 Separation 892
39.4 Recent Membrane Developments for CO2 Capture 893
39.4.1 General Criteria for Membrane Design 893
39.4.2 Perovskite Membranes for Selective O2 Permeation 895
39.4.2.1 Co–Containing Perovskites 895
39.4.2.2 Co–Free Perovskites 901
39.4.2.3 Dual–Phase Membranes 902
39.4.3 Perovskite Membranes for Selective H2 Permeation 904
39.4.3.1 Ce–Containing Perovskites (Cerates) 904
39.4.3.2 Dual–Phase Metal Cerates: Cermets 905
39.4.3.3 Ce–Free Formulations 909
39.4.4 Molten Carbonate/Perovskite Membranes for Selective CO2 Permeation 910
39.5 Conclusions and Perspectives 913
Glossary 915
Greek Symbols 915
Subscripts 915
Acronyms 915
References 916
Index 929
Vasile I. Parvulescu is Director of the Department of Organic Chemistry, Biochemistry and Catalysis at the University of Bucharest, Romania. He received his PhD in Chemistry from the University of Bucharest for a work on the selectivity of bi- and multi-metal catalysts in hydrogenation of aromatic hydrocarbons. After several years as senior researcher at the Institute for Non-Ferrous and Rare Metals in Bucharest, he rejoined the University of Bucharest in 1992 where he became full professor in 1999. His current interest concerns the study of heterogeneous catalysts for green and fine chemistry as well as for environmental protection. He authored more than 240 papers, 25 patents and 4 books.
Serge Kaliaguine is Professor in the Department of Chemical Engineering at the University of Laval in Quebec, Canada. He has developed a strong expertise in the fields of zeolites and mesoporous molecular sieves and perovskites and other mixed oxides for which he developed a novel reactive grinding technology for industrial applications. He is also involved in the preparation of composite proton conducting membranes. Serge Kaliaguine is co-author of more than 300 peer-reviewed publications in the field of applied chemistry, chemical engineering and polymer chemistry.
Wilfrid Prellier is Senior CNRS Researcher in the CRISMAT Laboratory at the University of Caen, Fran
Date de parution : 02-2016
Ouvrage de 1056 p.
17.5x24.9 cm
Disponible chez l'éditeur (délai d'approvisionnement : 12 jours).
Prix indicatif 407,00 €
Ajouter au panierThème de Perovskites and Related Mixed Oxides :
Mots-clés :
perosvskite