Multi-scale Quantitative Diagenesis and Impacts on Heterogeneity of Carbonate Reservoir Rocks, 1st ed. 2017 Advances in Oil and Gas Exploration & Production Series
Auteur : Nader Fadi Henri
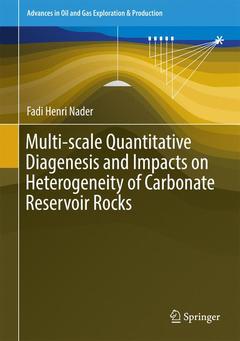
i
TABLE OF CONTENTS
General Summary of Scientific/Academic Activities xvii
Chapter 1: Introduction
1.1. Diagenetic realms 1
1.2. Porosity and diagenesis 4
1.3. Quantitative diagenesis 7
1.4. Numerical modelling of diagenesis 9
1.5. Objectives and way forward 13
Chapter 2: Characterization of diagenesis
2.1. State of the art (Characterization of diagenesis) 15
2.1.1. Fieldwork 16
2.1.2. Petrography 18Pre-microscopic observations 18
Microscopic observations 19
2.1.3. Geochemistry 20
Major and trace element analyses 20
Stable oxygen and carbon isotopic analyses 21
Strontium isotope analyses 21
2.1.4. Mineralogy 22
IFPEN protocol for XRD 22
Electron microprobe 23
2.1.5. Fluid inclusions 24
Microthermometry 24
Crush-leach analyses 24
2.1.6. Integrated techniques for building conceptual models 252.2. Future perspectives 27
2.2.1. Clumped oxygen isotopic analyses 27
2.2.2. Mg isotopic analyses 28
2.2.3. 3D porosity 30
Micro-CT setup and 3D-image reconstruction 31
Building an equivalent pore network from the actual pore space 31
Numerical simulation of mercury intrusion and permeability 34
ii
2.3. Discussion and personal contribution 35
2.3.1. General discussion 35
2.3.2. Personal contribution 37
Analytical methods: Dolomite stoichiometry assessment 37Dolomitization 38
Sandstone diagenesis 39
Source rocks 39
2.4. Advancement in characterization of diagenesis 41
2.5. Samples of published articles in peer-reviewed journals 43
Chapter 3: Quantitative diagenesis
3.1. State of the art (Quantitative diagenesis) 99
3.1.1. Petrography – Plug/Sample scale 99
3.1.2. Petrography – Reservoir scale 102
3.1.3. Mineralogical analyses (X-Ray Diffraction) 106
3.1.4. Geochemical analyses – Thi
n section scale 1093.1.5. Geochemical analyses – Reservoir scale 110
3.1.6. Geochemical analyses – Basin scale 112
3.1.7. Fluid inclusion analyses – Petroleum systems 114
3.1.8. 3D Scanning (CT and micro-CT) and image analysis 117
3.2. Future perspectives 121
3.2.1. Remote sensing and photogrammetry 121
3.2.2. Integrated data analysis tools 122
3.2.3. Pore space models 123
3.3. Discussion and personal contribution 125
3.3.1. General discussion 125
3.3.2. Personal contribution 128
Quantitative analytical methods 129Fluid inclusion analysis 129
Integrated petrographic and petrophysical data analyses 129
Micro-CT and image analyses 129
3.4. Advancement in quantitative diagenesis 131
3.5. Samples of published articles in peer-reviewed journals 133
iii
Chapter 4: Numerical modelling of diagenesis
4.1. State of the art (Numerical modelling
of diagenesis) 1814.1.1. Geometry-based modelling 181
Karst networks modelling 181
Fracture-related hydrothermal dolomite mod
elling 1854.1.2. Geostatistical modelling 188
Methodology: Geostatistical parameterization steps 188
Methodology: Geostatistical simulation methods 191
Case study: Geostatistical modelling of the Upper Jurassic Arab D reservoir heterogeneity, Offshore Abu Dhabi, United Arab Emirates (Morad, 2012) 197
Alternative workflow for geostatistical modeling of the Arab D reservoir heterogeneity 215
4.1.3. Geochemical modelling 220
0D geochemical modelling: Dissolution/Precipitation rates 220
2D geochemical reactive transport modelling: Reflux dolomitization 223
3D geochemical reactive transport modelling: Compactional dolomitization 228
4.2. Future perspectives 2334.2.1. Geometry-based modelling 233
4.2.2. Geostatistical modelling 233
4.2.3. Geochemical modelling 234
4.2.4. Towards integraded geomodels 235
4.3. Discussion and personal contribution 237
4.3.1. General discussion 237
4.3.2. Personal contribution 241
Geometry-based modelling 241
Geostatistical modelling 242
Geochemical modelling 242
4.4. Advancement in numerical modelling of diagenesis 245
4.
5. Samples of published articles 247 Chapter 5: Petroleum systems and basin evolution
5.1. The Levant Basin 283
5.2. State of the art (petroleum systems and basin modelling) 2905.2.1. Forward stratigraphic modelling 290
Methodology (DionisosFlowTM) 291
Levant Basin forward stratigraphic model 293
iv
5.2.2. Basin modelling 299
Methodology (TemisFlowTM) 300
Lithofacies transfer from DionisosFlowTM to TemisFlowTM 301
Levant Basin model 309
Synopsis 3165.3. Future perspectives 317
5.3.1. Towards integrated stratigraphic basin models 317
5.3.2. Towards integrated basin/reservoir models 319
5.4. Discussion and personal contribution 321
5.4.1. General discussion 321
5.4.2. Personal contribution 324
Stratigraphic modelling 324
Petroleum systems modelling 324
Structural modelling 325
5.5. Advancement in numerical integrated modelling 327
5.6. Samples of published articles in peer-reviewed journals 329
Chapter 6: Conclusions and general perspectives6.1. Characterization techniques and workflows 374
6.2. Quantitative techniques and workflows 375
6.3. Modelling techniques and workflows 376
6.4. Integrated modelling workflows 377
6.5. Way forward and research strategy 378
References 381
Extended curriculum vitae 399
v
LIST OF FIGURES
Figure i. From conceptual to numerical modelling of diagenesis, quantifying diagenetic phases remains essential…………………………………………………………………………………………….... xvi
iiChapt
er 1: IntroductionFigure 1- 1. Schematic representation of major diagenetic realms, related processes and resulting products in a carbonate platform.…………………………………………………………………...…………….... 1
Figure 1- 2. Field photograph showing the Lower Cretaceous microbialithes of the Qishn Formation in Wadi Baw (Oman) during a field visit with Petrobras (in 2007)…………………………………………... 3
Figure 1- 3. Diagenesis occurs in depositional and erosional environments with distinct spatiotemporal zones: Eogenetic, Mesogenetic and Telogenetic……………………………………………………………. 4
Figure 1- 4. Schematic illustration of porosity evolution during diagenesis: progressive dissolution (from mould to vug) and porosity reduction by cementation……………………………………………………… 5
Figure 1- 5. Porosity 3D network building based on micro-CT and image analyses (from De Boever et al., 2012)…………………………………………………………………………………………………. 6
Figure 1- 6. Integrated techniques for constraining flow properties (porosity and permeability) dur
ing dissolution of dolomite and successive anhydrite cementation of a reservoir rock…………………. 6Figure 1- 7. Photograph showing the extent of hydrothermal dolomites (dark brown) in platform carbonates (Ranero, northern Spain)…………………………………………………………………………….. 7
Figure 1- 8. 3D cube of a typical Jurassic Arab C dolostone sample (Middle East), which have been scanned with micro-CT at resolution of 1.5 μm………………………………………………………………. 8
Figu
re 1- 9. Illustrations showing subsampling schemes for determining the Representative Elementary Volume (REV)………………………………………………………………………………………………... 8Figure 1- 10. Geometry-based modelling of the fracture related Ranero hydrothermal dolomites (northern Spain)………………………………………………………………………………………………… 10
Figure 1- 11. Results of geostatistical stochastic joint simulations of sedimentary facies and diagnetic imprints of the three stratigraphic units in the Madison Formation, Wyoming – USA………………………. 11
Figure 1- 12. Results of reactive tran
sport modelling of reflux dolomitization showing the volumetric evolution (in %) of dolomite, anhydrite and porosity………………………………………………………….. 12Chapter 2: Characterization of diagenesis
Figure 2- 1. Map showing dolomite occurrence and fracture (fault) lineaments in Cretaceous platform carbonate rocks exposed in Ranero (NE Spain)………………………………………………………………… 16
Figure 2- 2. Out-crop scale petrographic observations on the world class fault-associated dolomites in the Ranero, Pozalagua quarry/auditorium (NE Spain)…………………………………………………... 17
vi
Figure 2- 3. Stained polished, etched slab of dolomite from the Ghalilah Formation (Upper Triassic) in Ras Al Khaimah (UAE)……………………………………………………………………………………… 18
Figure 2- 4. SEM backscattered electron image (left) and cluster analysis of electron microprobe map (right), as well as mean structural formula for two different dolomites………………………………………... 2
3Figure 2- 5. Proposed sequence of diagenetic phases (paragenesis) for the dolostones of the Marjaba HDT front (central, Lebanon)……………………………………………………………………………………. 25Figure 2- 6. Examples of conceptual dolomitization models and their schematic geometries and extent… 26
Figure 2- 7. Interpolated (solid) line of Δ47 values (in ‰) and corresponding temperatures (in K) for inorganic and organic carbonates (from Eiler, 2007)…………………………………………………………... 28
Figure 2- 8. Theoretically predicted Mg isotope fractionation factors (from Li et al., 2012 and references therein)……………………………………………………………………………………………….. 29
Figure 2- 9. Extracted macro-pore network for the Estaillades carbonate rock standard. ………………… 30
Figure 2- 10. (A) 3D grey scale view of micro-CT scan (2000x1000x1000 pxls) of a typical Jurassic Arab C dolostone (Middle East). (B) Grey level histogram (De Boever et al., 2012)……………………….. 32
Figure 2- 11. Equivalent network building of the actual pore
structure of a typical Jurassic Arab C dolostone (Middle East)………………………………………………………………………………………… 33Figure 2- 12. Simulated and measured mercury injection capillary pressure curve of the actual pore structure of a typical Jurassic Arab C dolostone (Middle East)………………………………………………….. 34
Figure 2- 13. Burial model for a well intercepting Khuff equivalent reservoirs (Kangan Formation) in the Salman field, offshore Iran (Peyravi et al., 2014 )………………………………….……………….. 36
Chapter 3: Quantitative
diagenesisFigure 3- 1. Scanning electron microscope (SEM) photomicrographs coupled with nuclear magnetic resonance (NMR) T2 distribution graphs of two typical carbonate rocks ……………………………………… 100
Figure 3- 2. Correlation cross-section (constructed by EasyTraceTM software based on petrographic analyses of thin sections) ………………………………………..……………………………………………….. 103
Figure 3- 3. Statistical analyses of well data based on 10000 thin sections (and samples) from 13 wells (Arab D, Midd
le East).………………………………………………………………………………………… 104Figure 3- 4. Proportional maps showing: (i) the dolomite distribution (A: Wackestone-Packstone; B: Packstone-Grainstone; C: Mudstone-Wackestone); and (ii) the relative abundance of the syntaxial calcite overgrowth cement (D: wackestone-Packstone; E: Packstone-Grainstone; F: Mudstone-Wackestone) in the Arab D member of an investigated oilfield offshore Abu Dhabi (UAE)…………………………………….. 105
Figure 3- 5. XRD and Rietveld diagram showing the quantitative assessments of calcite, dolomite and anhydrite in a Triassic carbonate rock from the French Jura…………………………………………………... 106
Figure 3- 6. Cross-plot diagram of dolomite stoichiometry (%Ca in dolomite) versus dolomite cell parameter (c in Å) featuring data from various sources…………………………………………………………… 107
Figure 3- 7. Unit cell dimensions of Upper Muschelkalk and Lettenkohle dolomites…………………….. 108
Figure 3- 8. Spectral analysis
and resulting mineralogical mapping of a sandstone sample from the Lower Cretaceous Upper Mannville Formation (Alberta, Canada)…………………………………………. 109vii
Figure 3- 9. Detailed geological map of the Marjaba dolostone front with discordant geometry and its surrounding limestone host-rock (central Lebanon; Nader et al., 2007)……………………………. 110
Figure 3- 10. Fe and Mn concentration contour maps (A) and cross-plot (B) for the Marjaba HDT front (central Lebanon; concentrations in ppm)……………………………………………………………………. 111
Figure 3- 11. Schematic illustration representing the typical fracture-filling cement stratigraphy………… 113
Figure 3- 12. Calculated oxygen stable isotopic composition ranges of the ‘parent’ diagenetic fluids (expressed in δ18O VSMOW) for dolomite and calcite cement phases………………………………………..… 113
Figure 3- 13. Workflow for dating unaltered (preserved, A and D) and re-equilibrated (B and E) fluid water (aqueous) and petroleum inclusions…………………………………………………….…………… 116
Figure 3- 14. Examples of training i
mages of carbonate rock textures for constructing 3D models of pore space (with evolving diagenesis)…………………………………………………………………………… 117Figure 3- 15. Calculation of porosity% for increasing volumes to investigate the representative elementary volume of the studied sample…………………………………………………………………….….. 119
Figure 3- 16. 2D-grey scale view of CT scan of a typical dolomitized grainstone………………………… 119
Figure
3- 17. Integrated workflow for reservoir models based on 3D photogrammetry of outcrop analogues (from Schmitz et al., 2014)…………………………………………………………………………... 121Figure 3- 18. Hyperspctral image analysis and interpretation of the main face of the Pozalagua Quarry (Ranero, NE Spain; from Kurz et al., 2012)…………………………………………..……………………….. 122
Figure 3- 19. Rock-Eval pyrolysis results for identifying and quantifying carbonate species)…………….. 127
Chapter 4: Numerical modelling of diagenesis
Figure 4- 1. An example of a 3D speleological map of underground karstic networks (Llueva cave, Spain) with Survex and supported by Therion software packages………………………………………………. 182Figure 4- 2. Improved workflow for ODSIM of karstic networks…………………………………………. 183
Figure 4- 3. Simulated envelope of a karstic network skeleton obtained by taking into account the bedding plane (H1) – a stratigraphic feature, a fault (F1) – a structural feature, and an ‘attraction level’ (water-table) coinciding with the lowest conduits………………………………………………………...……….. 184
Figure 4- 4. Top views of the Ranero model (6000 x 2000 m) showing the two study areas (outlined in black) and the distribution of dolomites (brown) within the original sedimentary facies………………..… 185
Figure 4- 5. Based on field data and conceptual models, the Ranero fault-related dolomites can be further constrained by applying a distance decreasing control for the dolomite occurrence along fau
lts/fractures with increasing depth from a preferential horizon……………….………………………………….. 186Figure 4- 6.
Dolomite distribution simulation of the Ranero model (6000 x 2000 m) taking into account the NW-SE faults and the vertical geometry constraining factors……………………………………………. 187Figure 4- 7. The principle of computing a vertical proportion curve (VPC)………………………….……. 189
Figure 4- 8. Schematic representation of the computation of VPCs and VPMs……………………….…… 189
viii
Figure 4- 9. An example of a variogram showing its characteristic parameters…………………………… 190
Figure 4- 10. Influence of variogram model type (A: Exponential; B: Spherical; and C: Gaussian) on the resulting distribution of modelled properties………………………………………………..………. 191
Figure 4- 11. (A) The principles of the Sequential Indicator Simulation (SIS). (B) The way categorical variables are transformed into indicator functions……………………………………………………………...192
Figure 4- 12. The principle of truncated Gaussian approach……………………….…
……………………. 193Figure 4- 13. The plurigaussian method and related parameters……………………………………...……. 194
Figure 4- 14. The multiple-point geostatistics applied on channels…………….………………………….. 195
Figure 4- 15. Schematic depositional model of the Arab D and C members……………………….……… 197
Figure 4- 16. Three well-logs from southeast outer ramp to the northeast inner ramp across the investigated field, showing the rock texture dist
ribution of the investigated parasequence set………………….. 198Figure 4- 17. Simplified map showing the investigated field (~20 x25 km) at the PS3 interval, the extent of the depositional facies, as well as the locations of wells………………………………………………… 199
Figure 4- 18. Photomicrographs (PPL) displaying characteristic lagoon microfacies in PS3……………… 200
Figure 4- 19. Photomicrographs (PPL) displaying examples of shoal microfacies in PS3………………… 200
Figure 4- 20. Photomicrographs (PPL) displaying characteristic outer shoal microfacies in PS3…….…… 201Figure 4- 21. Photomicrographs (PPL) displaying characteristic outer ramp microfacies in PS3…………. 201
Figure 4- 22. Cross-plots of bulk porosity (%) versus log-permeability analysed based on the abundance of SCOC and dolomite% within the PS3…………………………….…………………………………. 203
Figure 4- 23. Geostatistical modeling workflow for the distributions of rock textures (in three classes: grainy, packstone, muddy), the SCOC (in four classes: absent, rare, common, abundant), dolomite (%) and porosity (%) in PS3………………………………………………….………………………………. 204
Figure 4- 24. (Left) Depositional facies map showing the location of the wells (hard data) across the investigated field. (Right) Computed vertical proportion curves (VPCs) representing the vertical rock textures distributions in each of the ten wells…………………………...……………………………………. 205
Figure 4- 25. Property map showing the extent of the depositional
environments (i.e. areas)………...…… 206Figure 4- 26. Co
nstrained proportion matrix (VPM) for rock textures in PS3……………………..………. 206Figure 4- 27. Top view of the modelled rock textures distribution in PS3…………………………………. 207
Figure 4- 28. (Left) Depositional facies map showing the location of the wells (hard data) across the investigated field. (Right) Reported well-logs data representing the vertical SCOC abundance distributions in each of the ten wells………………………………………………………………………………………….. 208
Figure 4- 29. Property map for constraining the distribution of SCOC in PS3……………………….……. 209
Figure 4- 30. Constrained proportion matrix (VPM) for SCOC in PS3…………………...……………….. 210
Figure 4- 31. Top view of the modelled SCOC distribution in PS3……………………………...………… 211
Figure 4- 32. Top view of the modelled dolomite distribution in PS3………………………….………….. 212
ixFigure 4- 33. View of the porosity attribution in PS3, based on the modelled distributions of rock textures, SCOC and dolomite as well as the diagenetic drivers rules…………………….…………………… 213
Figure 4- 34. View of the permeability attribution in PS3, based on the modelled distributions of rock textures, SCOC and dolomite as well as the diagenetic drivers rules…………………………………………. 214
Figure 4- 35. Geostatistical modelling workflow for the distributions of facies (rock textures in three classes: grainy, packstone, muddy) by means of plurigaussian method……………………………
...……….215Figure 4- 36. Results of the two Gaussian simulations
(A: G1; B: G2) across the study area for the rock textures (facies)………………………………………………………………………………..……………… 216Figure 4- 37. Distributions of rock textures (facies; A), porosity in % (B), SCOC (C) and permeability in mD (D) based on geostatistical modelling involving plurigaussian, FFT-MA, collocated cokriging methods for the PS3 interval (Upper Jurassic Arab D Member)…………
……………….………………………. 219Figure 4- 38. General characteristics of reflux dolomite model………………………………………...….. 223
Figure 4- 39. Conceptual model of reflux dolomitization, including both processes of replacement of calcite and precipitation of dolomite cement (overdolomitization)……………………………………………… 225
Figure 4- 40. Simulation results of reflux dolomitization 2D geochemical RTM with the original brines at 50°C and in three time steps (0.1, 0.2 and 0.3 m.y. after the start of fluid injection)…………………...…. 226
Figure 4- 41. A typical example of sensitivity analyses demonstrating the influence of the reactive surface areas (102 and 104 cm2/g) on the modelled dolomite and anhydrite precipitations as well as porosity; from Jones and Xiao (2005)………………………………………………………………..…………………….. 227
Figure 4- 42. Simplified map and cross-section showing the paleogeographic configuration at the Jurassic times of the Po Plain and the southern Alps (from Consonni et al., 2010)………………………………… 228
Figure 4- 43. Basin (burial) modelling results across the Canonica basin and Malossa Paleohigh (Po Plain, Italy; from Consonni et al., 2010)………………………………………………………………………..… 229Figure 4- 44. Results of the 3D geochemical reactive transport model (RTM; with TOUGHREACT code) covering the Malossa Paleohigh…………………………………………………...………………… 230
Figure 4- 45. Results of the 3D geochemical reactive transport model of the Malossa Paleohigh highlighting the impact of two different configurations of faults/fractures………………………...…………………. 231
Figure 4- 46. Towards integrated basin/reservoir geomodels……………………………...……………….. 235
Figure 4- 47. Results of geochemical RTM simulations achieved by ArXim-CooresTM representing the formation of hydrothermal dolomite fronts (dolomite, anhydrite and porosity%)………………….. 240
Chapter 5: Petroleum systems and basin evolution
Figure 5- 1. Schematic representation of major diagenetic processes within basin-scale and/or reservoir-scale evol
ution frameworks……………………………………………………...………………………… 281Figure 5- 2. Schematic map showing the Levant Basin and the major oil/gas fields in the East-Mediterranean region and northern Arabia (from Nader, 2014a)……………………………………………………. 282
Figure 5- 3. Petroleum systems model for onshore and offshore Lebanon showing potential source rocks, migration pathways, plays and seals (slightly modified from Nader, 2014b)……………….………. 284
x
Figure 5- 4. Chronostratigraphic chart showing observed
onshore sedimentary facies and inferred offshore facies in Lebanon (based on seismic interpretation)…………………………………….………………….. 285Figure 5- 5. Un-interpreted and interpreted south-north 2D seismic profile offshore Lebanon (courtesy of PGS), showing the structure of the Levant margin…………………………………………………………. 286
Figure 5- 6. An example of west-east oriented 2D seismic profile across the Levant Basin offshore northern Lebanon……………………………………………………………………………………………… 287
Figure 5- 7. (A) 2D seismic profile showing interpreted facies associated to the Oligo-Miocene deepwater depositional settings offshore northern Lebanon, with channel incisions and fan lobe (turbidite) deposits (Hawie, 2014). (B) Amplitude map of at the Upper Miocene horizon showing the extent of the fan lobe (turbidite) complexes offshore norther Lebanon (from Fürstenau et al., 2013)………………...…… 288Figure 5- 8. Field photograph of the organic-rich Campanian lime-mudstone facies exposed in the Chekka quarries (Cimenterie Nationale sarl.) in northern coastal Lebanon………………………………..… 289
Figure 5- 9. Conceptual model proposed for the eastern margin of the northern Levant Basin………...…. 290
Figure 5- 10. Input parameters and sediment accommodation calculation with DionisosFlowTM……...….. 292
Figure 5- 11. Constraining data for the forward stratigraphic model based on regional studies, seismic interpretation, fieldwork, and well correlations………………………………………………….….. 293
Figure 5- 12. Coverage
of the forward stratigraphic model for the northern part of the Levant Basin…..... 294Figure 5- 13. Schematic block-diagrams illustrating the Miocene depositional environments for the Levant Basin and its eastern margin………………………………………………………...……………….. 295
Figure 5- 14. Results of carbonate production modelling at the margin and in the basin based on the best-fit model (from Hawie et al., in press)……………………………………..…………………………… 296
Figure 5- 15. Results of the Levant Basin best-fit stratigraphic model (from Hawie et al., in press)…….... 297
Figure 5- 16. Best fit simulation for the Levant Basin stratigraphic filling between 16 and 6Ma…………. 298
Figure 5- 17. Northward view of the Levant basin model with simplified lithological facies…………...… 299
Figure 5- 18. TemisFlowTM Map Editor depth map to the top of the Triassic rock series………………..... 300
Figure 5- 19. Illustration of
the followed workflow to transfer the facies distribution resulted from the DionosisTM stratigraphic model into the TemisFlowTM model………………………...…………….. 301Figure 5- 20. Bathymetry map (in meters) of present day seabed and onshore surface (Top Pliocene – Quaternary)………………………………………………………..…………………………………. 302
Figure 5- 21. Thermal calibration in based on well data and surface exposed Cretaceous rocks……….…. 304
Figure 5- 22. Basal heat flow variation through time for the six pseudo wells within the Levant Basin…...
305Figure 5- 23. Transformation ratio map of the Permian Type III source roc
ks across the Levant Basin and margin…………………………………………………………………………………...…………… 309Figure 5- 24. Transformation ratio history of the Permian source rocks at the six pseudo wells…….…….. 309
Figure 5- 25. Transformation ratio map of the Kimmeridgian source rocks across the Levant Basin and margin (Bou Daher et al., submitted)…………………………………………………………….………….. 310
Figure 5- 26. Transformation ratio history of the Kimmeridgian source rocks at the six pseudo wells….… 310xi
Figure 5- 27. Transformation ratio map of the Neocomian source rocks across the Levant Basin and margin (Bou Daher et al., submitted)………………………………………………………………………... 311
Figure 5- 28. Transformation ratio history of the Neocomian source rocks at the four pseudo wells….….. 311
Figure 5- 29. Transformation ratio maps of two Campanian source rocks with distinct activation energies across the Levant Basin………………………………………………………………………..……………. 312
Figure 5- 30. Transformation ratio history curves of the two distinct Campanian source rocks across the Levant Basin…………………………………………………………………………………………...…….. 312
Figure 5- 31. Palaeogeographic map of the Levant region showing depositional environments in the Late Cretaceous times (Bou Daher et al., submitted)…………………………..…………………………. 313
Figure 5- 32. EW section across the constructed model showing the calculated vitrinite reflect
ance at present day (Bou Daher et al., submitted)………………….………………………………………………… 315Figu
re 5- 33. Future perspectives for integrated stratigraphic-structural and fluid flow geomodels……..... 317Figure 5- 34. Plot of activation Energy (E), preexponential factor (A), and error function, for one of the analyzed samples (12/134), showing the different possible E and A couples for a minimum error function… 318
Figure 5- 35. From left to right these are the P10, P50, and P90 transformation ratio maps using one of the analysed soure rock samples…………………………………………………………………...……. 318
Chapter 6: Conclusions and general perspectives
Figure 6- 1. Proposed future operational workflow for tackling diagenesis: (A) Conceptual studies of diagenesis – for example hydrothermal or high temperature dolomitization (HTD; Nader et al., 2004, 2007); (B) Quantitative diagenesis methods – e.g. micro-computed t
omography (micro-CT) image analyses (De Boever et al., 2012); and (C) Numerical simulations of diagenetic processes such as reactive transport modelling of dolomitization (e.g. Consonni et al., 2010)……………………………………………. 373Figure 6- 2. My research strategy on the general theme of “multi-scale integrated numerical modeling of diagenesis” for the coming three years (until 2018). Two parallel clusters of programmes are indicated (Diagenesis and Geological models). Principal cross-cluster themes are also indicated (upscaling, REV; scales and methods; case st
udies and validation). The regional expertise and related academic projects; such as supervising PhD projects are also listed per cluster.…………………………………..……. 379xii
xiii
LIST OF TABLES
Chapter 2: Characterization of diagenesis
Table 2- 1. Total list of material used throughout a typical diagenetic study (Nader, 2003)………………. 17
Chapter 3: Quantitative diagenesisTable 3- 1. Various lithological and textural categories for describing carbonate microfacies ……………. 101
Table 3- 2. Categories for describing cement in microfacies (thin sections)…………………………….… 101
Table 3- 3. Categories for describing reservoir properties in microfacies (thin sections)………………….. 102
Chapter 4: Numerical modelling of diagenesis
Table 4- 1. The quantitative impact of diagenesis on reservoir quality for the various depositional facies and rock textures in PS3……………………………………………………………………..…………… 202
Table 4- 2. The simulation method and variogram values for the rock textures distribution of PS3………. 207
Table 4- 3. The simulation method and variogram values for the SCOC distribution of PS3…………..….. 210
Table 4- 4. Variogram model for the
porosity (FFT-MA) simulations……………………...……………… 217Table 4- 5. Parameters for the collocated cokriging simulations of the SCOC distribution in packstone rock-textures across the study area, and constraints for the variogram model…………….……………… 217
Table 4- 6. Para
meters for the collocated cokriging simulations of the SCOC distribution in grainstone rock-textures across the study area, and constraints for the variogram model……………………...…….. 217Table 4- 7. Applied conditional formulas for calculating permeability values based on simulated porosity per rock texture (facies) taking into account the SCOC distribution…………..……………………… 218
Table 4- 8. Geochemical compositions of the fluids (associated to dolomite dissolution and anhydrite precipitation) used in reaction path calculations……………………………………….……………. 221
Table 4- 9. Quantitative diagenesis of three samples from the Arab Formation (Abu Dhabi – UAE) by means of micro-CT image analyses and XRD (from De Boever et al., 2012)…………..…………………….. 222
Table 4- 10. Sample chemical and physical input parameters for reaction path simulations. In gray: values of input parameters that were varied for a sensitivity study………………………………….………… 222xiv
Chapter 5: Petroleum systems and basin evolution
Table 5- 1. Results of bulk rock analysis with Rock-Eval6 for selected source rock samples………….….. 306
Table 5- 2. Pure organic matter Rock-Eval6 results for selected source rocks samples after acid treatment and DCM extraction (from Bou Daher et al., submitted)……………………………………..………….. 306
Table 5- 3. XRD results pre- and post-acid treatment of source rocks……………………………….……. 307
Table 5- 4. Mean activation energies and frequency factors for se
lected source rock samples……….…… 308xv
DEFINITIONS
ArXim Open source program for multiphase speciation, equilibrium and reaction calculations between minerals, aqueous solutions and gases (EMSE and IFPEN).AvizoTM 3D analysis software application for exploring and understanding materials structures and properties, in a wide range of materials science research areas (FEI).
CobraFlowTM Software for performing geologically meaningful simulations using geostatistical algorithms (IFPEN and Centre of Geostatistics of the Paris School of Mines).
CooresTM (CO2 Reservoir Environmental Simulator), a research code designed to study CO2 storage processes from the well to the basin scale (IFPEN). It simulates multi-component three-phase and 3-D fluid flow in heterogeneous porous media. To take into account mineralogy changes, the transport model is coupled with ArXim.
DionisosFlowTM (Diffusion Oriented Normal and Inverse Simulation Of Sedimentation), a deterministic 3D multi-lithology stratigraphic modelling software that simulates depositional processes in a sequence of steps moving forward in time (IFPEN).
.EasyTraceTM A multi-disciplinary 1D data processing and editing tool, featuring advanced spreadsheets and a wide range of functionalities for geologists and geophysicists (IFPEN).
EOR Enhanced Oil Recovery.
FracaTM Software capable of characterizing, modelling and calibrating faults and fractures. It bu
ilds consistent fracture networks, constrained in 3D by seismic and geological attributes.GOCAD (Geologic
al Object Computer Aided Design), a software package used for building and update, geo-referenced 3D subsurface models (Gocad Research Group, Georessources UMR 7359, Géologie - Université de Lorraine).InterWellTM Software capable to analyse post, pre stack and 4D seismic data, to compute multi cubes seismic wavelet calibrated at wells and to build a priori seismic impedance cubes honouring wells and stratigraphic data (IFPEN).
JMicroVision A freeware designed to describe, measure, quantify an
d classify components of all kinds of images allowing to analyze high definition images of rock thin-sections (http://www.jmicrovision.com).MatlabTM A high level programming language and interactive environment capable of advanced numeric computation, data analysis and visualization, programming and algorithm development and application (Mathworks).
PHREEQ-C. A computer program designed to perform a wide variety of aqueous geochemical calculations. It implements several types of aqueous models and is capable of undertaking speciation, saturation-index, and transport calculations (USGS).
TemisFlowTM Basin modelling software package for assessing regionally controlled petroleum systems and basin evolutions. It calculates the generation, migration and accumulation of fluids (IFPEN).
xvi
Dr. habil. Fadi Henri Nader is an Engineer Sedimentologist at the IFP Energies nouvelles (formerly: Institut Français du Pétrole, IFP). He is working on diagenesis (fluid-rock interactions) and its impacts on reservoir properties (porosity/permeability) at the plug-, reservoir- and basin-scales. he has also launched several new research projects related to reflection seismic interpretations and modelling of frontier basins.
Reinforces basic principles with well documented case studies and scientific arguments
Maximizes reader insights into quantitative aspects of diagenesis and reservoir rocks characterization
Provides new, creative workflows to undertake reservoir characterization
Includes supplementary material: sn.pub/extras
Date de parution : 10-2016
Ouvrage de 146 p.
17.8x25.4 cm
Disponible chez l'éditeur (délai d'approvisionnement : 15 jours).
Prix indicatif 116,04 €
Ajouter au panier